Unraveling the secrets of planetary interiors
From crust to core, experimental petrologists, we decipher the geological history of Earth and other planetary bodies and the processes that lead to surfaces capable of supporting life.
Armed with the Earth and Planets Laboratory's state-of-the-art high-pressure facilities, our researchers simulate the intense pressures and temperatures found within a planet's mantle and core. By subjecting geological materials to these extreme conditions, we seek to understand the physical and chemical processes governing the evolution of planetary interiors.
Top Questions
For more than a century, the study of rocks and the minerals that form them has been a focus of Carnegie research. And for most of that hundred-plus years, biology seemed to have nothing whatsoever to do with Earth’s diverse non-living materials. That mindset began to change in the 1990s when a group of Geophysical Laboratory scientists considered possible roles of minerals in life’s chemical origins. And it didn’t take long for them to realize that rocks and life have been intertwined for the past 4 billion years of Earth history: minerals played key roles in life’s origins, and life has returned the favor as one of the most important influences in the formation of minerals. We now understand that the geosphere and biosphere have co-evolved.
Learn more“The key to understanding geochemical mass transfer in Earth is in tracing the movement of volatile-rich melts and fluids in the mantle and crust – melts that often leave an indelible chemical stain on the rocks they pass through," says Director Michael Walter.
Once Earth was fully formed and volatile element budgets for the whole planet effectively established, the next question is “how are volatile elements partitioned between its major reservoirs: the core, mantle, lithosphere, and exosphere (hydrosphere+atmosphere)?” How do they cycle through these reservoirs over the age of the planet? In what solid and melts phases are volatile elements stored and transported in Earth’s interior? Does deep mantle cycling of volatile elements moderate their abundances in the exosphere over geological time? What role does the core play as a volatile element holding tank and does it exchange volatile elements with the mantle? At EPL we are using a range of petrological, experimental, geochemical, and theoretical approaches to investigate these questions.
Learn more“The ability to make these measurements is crucial to developing reliable models of the internal structure of super-Earths up to eight times our planet’s mass,” Staff Scientist Yingwei Fei stated in a recent press release. “These results will make a profound impact on our ability to interpret observational data.”
Generally speaking, the Earth consists of a silicate mantle, a liquid iron-dominated outer core, and a solid iron-dominated inner core. We know this thanks to seismologists, who use earthquake waves like ultrasound to image the interior structures of our planet.
Research in experimental petrology and mineral physics is a key tool that allows us to determine the inner structure of our planet in more detail. Using high pressure and temperature experimentation, we can relate the chemistry and mineralogy to what we see in observed seismic profiles— from the surface to the center of the core.
Learn more“Understanding the introduction, transport, and storage of volatile elements in the interiors of terrestrial-like planets is profoundly linked to the nature of habitability and the sustainment of life," says EPL staff scientist Anat Shahar,
Volatile elements like carbon, hydrogen, oxygen, nitrogen, and sulfur are a cornerstone of planetary habitability yet it is unclear how the terrestrial planets obtained them and what their original budgets were. These elements are often mobile and readily exchange among reservoirs ranging from interstellar to sub-mm in scale.
At EPL we are seeking answers to the many questions relating to the inventories of volatile elements and how they are affected by a range of processes during planet formation.
Understanding how the deep Earth communicates with the Earth’s atmosphere and hydrosphere requires knowledge of the processes that connect the deep Earth to the surface, processes that invariably involve partial melting of mantle and crustal rocks.
How silicate melts are generated and migrate is strongly controlled by the very powerful role of even trace amounts of volatile elements. This is important because volatile-laden silicate melts are the primary means by which matter and energy move through the mantle and crust to Earth’s surface. Volatile-element-rich melts also are responsible for the most dangerous forms of explosive volcanism.
We know that the physical properties of silicate melts—like their density, viscosity, resistivity, and molecular diffusivity—are strongly controlled by the molecular structure of the melts themselves. Even small amounts of volatile elements can significantly modify melt structure and change these properties, often by many orders of magnitude. These changes are far in excess of what the variables of pressure or temperature typically provide. Nevertheless, our understanding of how volatile elements interact with melt structure is largely phenomenological such that a detailed understanding of volatile-melt interactions remains poorly understood. Ultimately, a complete understanding of the molecular structure of volatile-bearing silicate melts is essential.
Learn moreThe most basic features of heat transport from the top of the core to the top of the mantle are well understood: the highly conductive liquid outer core delivers as much heat to the base of the mantle as the mantle can absorb. The mantle, in turn, absorbs heat by conduction through its deepest layer, heat that is then transported by convection in all other regions of the mantle and ultimately to the lithosphere where it is released through volcanism and conduction.
The amount of heat leaving the core is a big deal because this feature largely controls the timing of inner core crystallization and generation of the magnetic field. The major unknowns that today’s researchers are investigating are focused on the lowermost layer of the mantle where conduction is dominant. What is the thickness of this layer? What is the rate of heat flow through this layer? What is the temperature difference across this layer? What is the temperature profile of the core?
We have developed a range of experimental and theoretical tools to interrogate these questions that include ultra-fast pulse-probe techniques to measure thermal conductivity in metals and silicate and latent-heat melting probes.
Learn more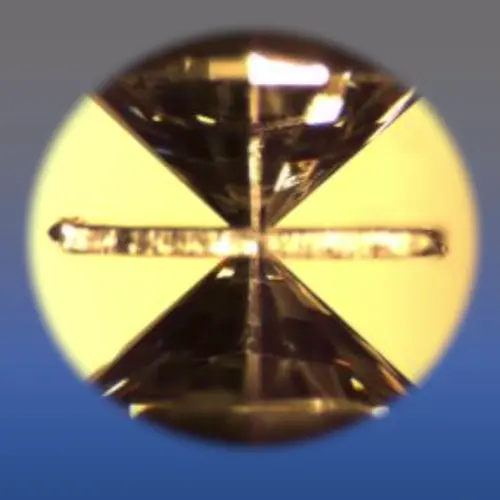
Tools of the Trade
Experiments at pressure and temperature conditions ranging from planetary crusts to cores are made using a wide range of tools, including 1 atm furnaces, cold-seal devices, piston cylinders, multi-anvil presses, laser-heated diamond anvil cells, and dynamic compression facilities. We analyze and characterize natural and experimental samples using our wide-ranging in-house capabilities, including SEM, EPMA, FIB, NMR, Raman, FTIR, and X-ray diffraction.
We use national and international synchrotron facilities to probe samples in situ at micron and sub-micron scales. Theoretical approaches utilize high-performance computing systems both on and off-campus.
All instrumentation