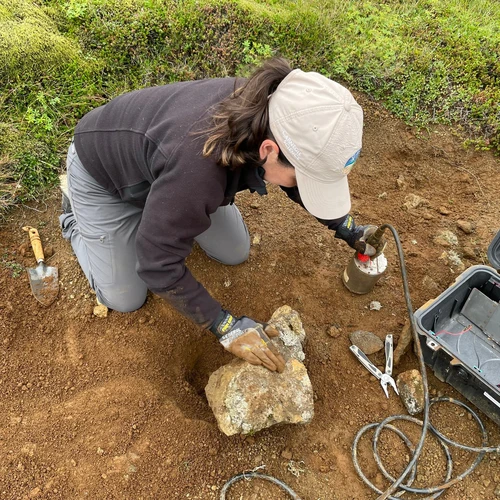
Illuminating the inner workings of our planet
Through fieldwork, monitoring, and advanced computation, our scientists have been global leaders in uncovering the secrets of Earth's dynamic interior for more than a century.
At the Earth & Planets Laboratory, we study the internal structure and dynamics of our planet and the physical processes that give rise to earthquakes and volcanic eruptions. By collecting data around the world, we study a wide variety of problems on many spatial and temporal scales. Studies range from mantle convection and subduction-zone dynamics to the formation and evolution of Earth’s magnetic field.
Our Top Questions
The geodynamo is the process by which Earth generates its magnetic field by convection in the liquid iron-rich outer core. Evidence supporting the existence of the ancient geodynamo extends back to at least 3.5 billion years ago—possibly as far back as 4.2 billion years, nearly the age of the Earth itself.
How has Earth maintained a dynamo-generated magnetic field for so long? No one knows for sure, but the answer is intertwined with how Earth has maintained a habitable surface for so long.
Staff Scientist Peter Driscoll is currently working on modeling the thermal evolution of the Earth to better constrain the timing of inner core solidification and the cooling rate of the interior, which is critical to understanding what was driving the geodynamo in the deep past.
Learn moreOne of the biggest challenges in volcanology is identifying and understanding eruption precursors (NASEM, 2017).
Staff Scientists Hélène Le Mével and Diana Roman use geophysical observations to study magmatic processes beneath volcanoes. By improving our understanding of volcano deformation, we will be better able to interpret future deformation patterns, including pre-eruptive deformation. Studies of volcanic seismicity and deformation are complementary and other observables such as volcanic gas flux and composition help forecast eruptive behavior.
Learn moreStudying the Earth’s interior is particularly challenging because we cannot see through rock. Or can we?
Carnegie scientists like Lara Wagner and Diana Roman follow on a long legacy set by emeritus Carnegie scientists David James, Selwyn Sacks, Alan Linde, and Paul Silver of collecting seismic data sets in order to image the interior of the Earth. However, when it comes to telling us what types of rocks are under our feet, progress has been limited by technology.
Learn moreThe start of plate tectonics is not well documented in the geological record but has likely been going on for at least the last 3.5 billion of the 4.5 billion years since Earth’s formation.
For a long time, scientists assumed that this difference between the crust and the deep mantle—seen in the detailed trace element chemistry of mid-oceanic ridge and ocean island basalts—was primarily caused by processes in the very early Earth combined with the extraction of the continental crust. However, scientists at Carnegie’s Earth and Planets Laboratory thought there was more at play—the formation and recycling of oceanic crust.
Learn moreMost earthquakes occur close to the Earth’s surface, down to about 70 kilometers. They happen when stress builds up at a fracture between two blocks of rock—known as a fault—causing them to suddenly slide past each other.
However, deeper into the Earth, the intense pressures create too much friction to allow this kind of sliding to occur and the high temperatures enhance the ability of rocks to deform to accommodate changing stresses. Though theoretically unexpected, scientists have been able to identify earthquakes that originate more than 300 kilometers below the surface since the 1920s.
New research published in AGU Advances provides evidence that fluids play a key role in deep-focus earthquakes—which occur between 300 and 700 kilometers below the planet’s surface.
Learn more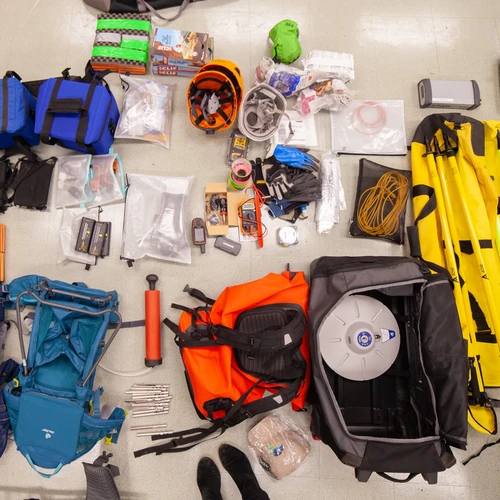
Tools of the Trade
We have deployed seismic networks in the western United States, Chile, Peru, Hawai'i, Italy, and Nicaragua. We are also actively involved in the development of cutting-edge next-generation instrumentation for observational geophysical research like gravimetry and quick-deploy seismometers. Computational geodynamics utilizes high-performance computing systems both on campus and the Carnegie Memex cluster.