Top Questions
By Richard Carlson, Staff Scientist Emeritus
Our Solar System started as a diffuse cloud of dust and gas at a density of a few hundred molecules per cubic centimeter and a temperature of about 10-15 degrees above absolute zero. Something caused that cloud to collapse, either the gravity of its own material or perhaps it was given a “push” by the shock wave from an exploding nearby star in the galaxy. As the cloud collapsed, gravity took over to gather most of the mass of the cloud into the central star, the Sun in our case. Because the molecular cloud was slowly rotating before the collapse, angular momentum caused a small percentage of the collapsing cloud to form a flat disk of material around the Sun.
The alignment of the planets along a single plane, the ecliptic plane, is good evidence of their formation from a disk of this nature.
Close to the central star, the disks were very hot—hot enough that most of the material was vaporized. As the disk eventually started to cool down, mineral grains began to condense, starting with those containing calcium, aluminum, and titanium that condense at the highest temperatures. Accumulations of these refractory grains, called calcium-aluminum inclusions (CAI) are found in some primitive meteorites and provide ages of 4.568 billion years—setting the start time of the planets in our Solar System.
On further cooling, the major silicates—olivine, and pyroxene—began to condense. Further away from the Sun, where temperatures were colder, various ices such as water and carbon monoxide also could condense. The boundary in the present asteroid belt between the relatively dry and rocky inner planets and the huge gaseous and icy outer planets can be thought of as a “snow line” where ices condensed only outside the snow line. The snow line concept provides a first-order explanation for why we have rocky and icy planets.
Digging deeper, our analyses of the neodymium and chromium isotopic composition of different types of meteorites along with rocks from Earth, Mars, and the Moon provided an early indication that the group of meteorites believed to come from further out in the Solar System—the carbonaceous chondrites—are made up of a different mixture of Solar System components than Earth and groups of meteorites thought to originate from the inner Solar System.
Other studies have confirmed and extended this distinction to arrive at the suggestion that Jupiter may have formed very early in the Solar System, opening up a gap in the disk, isolating the inner from the outer Solar System. If so, this could explain why the terrestrial planets are depleted not only in water but in other moderately volatile elements like the alkali metals and lead. Modeling the composition of Earth based on the various components found in meteorites can provide a reasonable match to Earth compositions on the assumption that Earth is made up primarily of the more refractory components in meteorites (CAIs and chondrules) with only a smaller role for the more volatile-rich matrix component seen in carbonaceous chondrites.
This model provides additional support for the idea that the early formation of Jupiter may have cut off the inner Solar System from the more ice-rich objects formed in the outer Solar System, leading them to be relatively deficient in water and other volatile components.
Learn more
The origin of Earth’s water is an open question and quite controversial. It really is part of a larger question about how Earth got its very volatile elements that include not just the hydrogen in water, but also carbon, nitrogen, and the noble gases (helium, neon, argon, krypton, and xenon) amongst others. Ultimately, any complete explanation must be able to reproduce both their elemental abundances and their isotopic compositions. It must also be compatible with what we know about how and on what timescales planetary systems form from the disk of gas and dust (protoplanetary disk) that surrounds a young star. In the case of the Solar System, the protoplanetary disk around the young Sun is generally referred to as the Solar Nebula.
Currently, the most favored explanation for where the Earth got its water is that it acquired it from water-rich objects (planetesimals) that made up a few percent of its building blocks. These water-rich planetesimals would have been either comets or asteroids. From what we know about comets, they are not a good match to either the elemental or isotopic composition of the Earth. It may also have been difficult to deliver enough comets to the Earth.
Water probably came from asteroids
At present, asteroids up to a few hundred kilometers across seem the most likely sources of most of Earth’s water, specifically the types of asteroid that dominate the outer asteroid belt between Mars and Jupiter. We have samples of some of these asteroids in the form of CM and CI carbonaceous chondrite meteorites. When they formed, these asteroids/meteorites contained water-ice, but radioactive heating caused the ice to melt and react with the anhydrous minerals to make clays. The meteorites also contain quite a bit of organic matter that contains hydrogen, carbon, and nitrogen – the hydrogen would have reacted to form water when the asteroids impacted the early Earth.
Noble gases even seem to be associated with the organics. The CM chondrites, in particular, give a reasonable fit to many aspects of Earth’s highly volatile elemental and isotopic compositions, although small amounts of cometary and Solar Nebula material produce an even better fit. Nevertheless, disagreements remain and there is much debate about whether these are due to some combination of (i) inaccuracies in estimates of the Earth’s volatile contents, (ii) storage of hydrogen and other volatiles in the Earth’s core, and (iii) loss of volatiles to space in the giant impacts that dominated the final stage of Earth’s formation.
Where do water-bearing asteroids come from?
If CM- and/or CI-like chondrites were the main sources of Earth’s water, where did they come from? It looks like they are representatives of a swarm of planetesimals that formed in the colder regions of the Solar Nebula beyond the orbit of Jupiter where water-ice-rich dust was plentiful, and they were scattered into the regions where Earth and the asteroid belt formed by a growing Jupiter.
Learn moreBy Larry Nittler, George Cody, Conel Alexander, & Dionysis Foustoukos
We know that complex organic molecules are abundant in space from telescopic observations of molecular clouds and protoplanetary disks, from spacecraft observations of comets and asteroids, and from laboratory analysis of meteorites and other extraterrestrial materials. Comets and asteroids delivered large amounts of such molecules, including life-essential amino acids, to the Earth as it was forming, perhaps providing the non-biological building blocks from which life was able to eventually emerge.
At the Earth and Planets Laboratory, Conel Alexander, George Cody, Dionysis Foustoukos, and Larry Nittler study organic material in meteorites and comet samples in order to learn how and where it formed and to better understand the original molecular inventory of Earth.
Primitive meteorites (those that come from asteroids that have experienced very little heating after their formation) contain up to several percent of their weight as organic carbon. Some of this is in the form of water-soluble molecules like amino acids, but most of it is in a more coal-like, complex material known as IOM (for insoluble organic matter).
George Cody uses the techniques of Nuclear Magnetic Resonance spectroscopy, synchrotron-based X-ray spectroscopy, and infrared spectroscopy to tease out the complicated molecular structure of meteorite IOM and infer possible formation mechanisms. His work has shown that the chemical nature of the IOM is consistent with formation in the Solar System from a chemical reaction series starting with the simple molecule formaldehyde, which is known to be present in the icy mantles of interstellar grains from which planetesimals ultimately accrete early in Solar System history. In a series of experimental studies, George Cody together with Dionysis Foustoukosalso probes how low levels of heating in asteroids modifies the molecular structure of IOM.
Conel Alexander and Dionysis Foustoukos study the average elemental and isotopic composition of organics in different types of meteorites. Interestingly, meteoritic organics, including IOM, show unusual isotopic characteristics; much of the material is highly enriched in the rare isotopes deuterium and nitrogen-15 (heavy forms of hydrogen and nitrogen, respectively). Such signatures can most easily be explained by chemical reactions occurring at very low temperatures and may point to a presolar origin of the organics (or their precursors) in the molecular cloud from which the Sun formed.
Furthermore, experimental studies have identified physicochemical processes that may control the evolution of these rare isotopes in altered IOM. Larry Nittler and Conel Alexander use high-resolution secondary ion mass spectrometry to look at very small-scale isotopic variations in meteoritic organics. Such work has found extreme variability in the isotopic composition of the organic matter on micrometer scales (figure).
By looking at both the bulk and micro-scale chemical variations within and between different meteorites, the EPL researchers hope to disentangle the effects that occurred on the meteorites’ parent asteroids (e.g. heating and interactions with liquid water) from the original properties of the organic matter to fully understand its origin, how it is modified during planet formation, and what eventual role it may have in the origin of life on Earth.
Learn more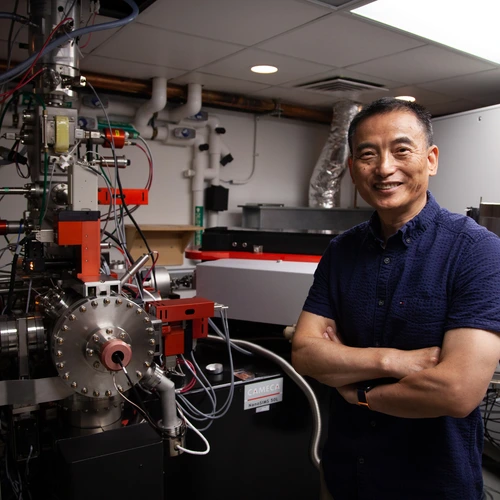
Tools of the Trade
We maintain six mass spectrometers for analyzing trace elements and isotope ratios at high sensitivity, high precision, and/or high spatial resolution. Our scientists share four electron beam instruments for the characterization of sample surfaces and elemental compositions, as well as for focused ion beam lift out for additional characterization such as transmission electron microscopy. We also utilize solid-state NMR, Raman, and FTIR spectroscopy to characterize natural and experimental samples.
All instrumention