Overview
Scientists simulate the high pressures and temperatures of planetary interiors to measure their physical properties. Yingwei Fei studies the composition and structure of planetary interiors with high-pressure instrumentation, including the multi-anvil apparatus, the piston cylinder, and the diamond anvil cell.
The Earth was formed through energetic and dynamic processes. Giant impacts, radioactive elements, and gravitational energy heated the planet in its early stage, melting materials and paving the way for the silicate mantle and metallic core to separate. As the planet cooled and solidified, geochemical and geophysical “fingerprints” developed as the mantle and core differentiated, magma solidified, and other early processes.
Yingwei Fei examines materials at high pressure and temperature in the lab. He is interested in phase transitions—what happens when materials change from liquid to solid to gas—what is behind elements separating and their melting relations, and chemical reactions and physical properties of these materials. His experimental research is used to interpret geochemical and geophysical observations of our planet and is applicable to geophysics, petrology, mineral physics, and planetary sciences.
Researchers in his lab use various high-pressure techniques. The Piston-cylinder simulates the upper mantle, while the multi-anvil device simulate the mantle. To conduct experiments at temperatures within the deep Earth, he uses various heating techniques included laser.
Fei received a BSc in geochemistry from Zhejiang University, China, and he obtained a Ph.D. in geochemistry from City University of New York. He was both a pre and postdoctoral fellow at Carnegie, then a fellow at the Norton Company before becoming a staff associate at Carnegie in 1991. In 1996 he joined the senior scientific staff.
Research
Planet differentiation could proceed through efficient liquid-liquid separation or by percolation of liquid metal in a solid silicate matrix, depending on the size and interior temperature of the planetary bodies. The percolation of liquid metal in the solid silicate matrix is likely a dominant process in the initial differentiation when the temperature is not high enough to melt the entire planetary body. The efficiency of percolation depends on the dihedral angle, determined by the interfacial energies of the solid-solid and solid-liquid interfaces.
Fei and colleagues simulate this process in the laboratory by conducting high-pressure and high-temperature experiments on mixture samples of iron alloy and silicate. They have developed a new imaging technique to visualize the distribution of liquid metal in the silicate matrix in three dimensions by a combination of focus ion beam (FIB) milling and high-resolution field-emission SEM imaging.
The new imaging technique provides precise determination of the dihedral angle and quantitative measure of the volume fraction and connectivity of the liquid phase [Fei, 2013].
The Earth and other terrestrial planets were differentiated through metal-silicate separation, forming iron-nickel metallic cores with dissolved light elements such as sulfur, oxygen, silicon, carbon, and hydrogen. Subsequent cooling led to the crystallization of the inner core of some planets.
Fei's aims to identify the light elements in the cores using an integrated approach, including element partitioning experiments, liquid metal percolation in molten or crystalline silicate matrix, and simultaneous measurements of density and sound velocities at high pressure and temperature. The ultimate goal is to establish comprehensive geochemical models of the planetary cores which are also consistent with the geophysical observations.
High-pressure synthesis has revolutionized the diamond industry and it continues to play important roles in searching for new super-hard materials. It has been demonstrated that pressure can enhance materials properties and lead to new phenomena and novel physical properties, which may be utilized to advance technology and design new materials. Fei has started to explore the role of pressure in materials synthesis, and established a new research avenue toward the synthesis of novel carbon-based super-hard materials at high pressure.
Fei and his team have successfully synthesized the first stishovite (a high-pressure form of silica) nanocrystals in multi-anvil high-pressure device. They were also successful in synthesizing new periodic crystalline mesoporous materials through high-pressure synthesis. Through nanocasting, they have made periodic mesoporous coesite, which is the first example of a periodic mesoporous high-pressure phase with unique physical properties. They have also explored the layering structure of graphane at high pressure and its transition mechanism to diamond at high pressure.
Mesoporous materials belong to the most important classes of materials due to their wide structural diversity and a broad range of applications including catalysis, separation, microelectronics, and drug delivery. The emphasis of the research is to explore the role of high pressure in synthesis to produce mesostructures of diamond and investigate the mechanisms of phase transformations at high pressure inside a mesostructure. Diamond is arguably the most technologically important high-pressure phase. Mesostructured composites of diamond could find applications as ultrahard materials. Diamond is known as biocompatible material and thus mesoporous forms of diamond could even have potential in drug delivery.
Read moreThe Earth’s core-mantle boundary (CMB) and inner core boundary (ICB) are the most important deep chemical boundaries that define the dynamics of the interior and control the generation of Earth's magnetic field.
The boundaries reset the element redistribution through metal-silicate differentiation and inner crystallization. Fei aims to understand the behavior of element partitioning at these boundaries using newly developed high-pressure and temperature techniques and state-of-the-art analytical tools that allow simulating the conditions of the boundaries and analyzing the chemical compositions of the coexisting phases in the recovered samples. The research will significantly advance our knowledge of Earth’s deep processes and experimental techniques to investigate element redistribution at deep chemical boundaries.
Read moreUsing innovative instrumentation and high-pressure techniques, Fei studies the thermal and density structures of planetary interiors.
Thermal Conductivity of Iron Alloys at High Pressure
The efficiency of heat transfer by conduction in a planetary core controls the dynamics of convection and limits the power available from the heat source. It also affects the thermal evolution of the planet.
Fei and colleagues have initiated a new research program that is aimed at measuring the electrical resistivity (inversely proportional to thermal conductivity through the Wiedemann-Franz law) of iron alloys using both multi-anvil and the diamond anvil cell techniques. This is complementary to their ongoing research on planetary cores, providing constraints on the heat budget and thermal structure of the interior.
Sound Velocity Measurements of Iron at High Pressure and Temperature
Seismic data are crucial for our understanding of the internal structure the Earth. However, we have limited lunar seismic data and no data on other terrestrial planets. Planetary seismic data will always be limited because of the limitation of resources and technology and their interpretation will heavily rely on laboratory measurements, particularly sound velocities and densities of mantle and core materials.
Phase relations and physical properties of iron play an important role in understanding planetary cores. In this research, Fei focuses on measurements of the sound velocity of fcc-Fe using the inelastic x-ray scattering (IXS) technique. Fei and his team have also established an experimental technique to measure the sound velocity of iron under simultaneous high pressure and temperature by combining inelastic x-ray scattering with the externally heated diamond-anvil cell.
CV
- Ph.D., City University of New York, Ph.D. 1989
- B.S., Zhejiang University, China, B.S. 1982
- 2018 Mineral name: Feiite
- 2013 Geochemistry Fellow of the Geochemical Society (GS) and the European Association of Geochemistry (EAG)
- 2010 Fellow of the American Geophysical Union
- 1999 Mineralogical Society of America Award
- 1999 Life Fellow of the Mineralogical Society of America
- 1992-1996 Norton Senior Fellow
- 1990 Hou Defeng Medal, Chinese Academy of Sciences
- 1989 Distinguished Scholar Dissertation Award, City Univ. New York
- 1996-present, Staff Scientist, Earth and Planets Laboratory, Carnegie Institution for Science
- 1991-1996, Associate Staff Member, Geophysical Laboratory, Carnegie Institution for Science
- 1992-1995, Norton Senior Fellow, Norton Company
- 1989-1991, Postdoctoral Fellow, Geophysical Laboratory, Carnegie Institution for Science
- 1988-1989, Predoctoral Fellow, Geophysical Laboratory, Carnegie Institution for Science
- 1982-1984 Institute of Geochemistry, Chinese Academy of Sciences
- American Geophysical Union
- Mineralogical Society of America
- Geochemical Society
Media and Talks
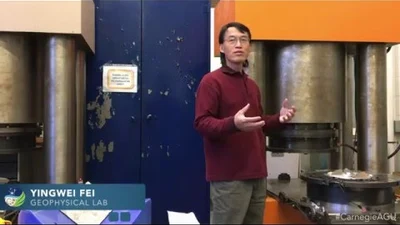
Yingwei Fei @ AGU 2015
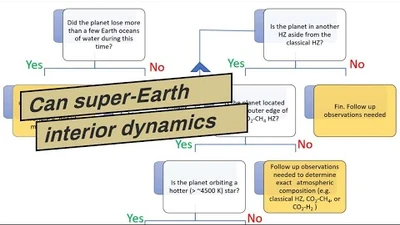
Can super-Earth interior dynamics set the table for habitability?
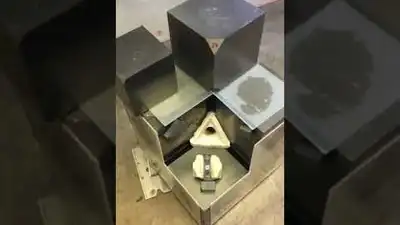
Walker Multianvil Press in Yingwei Fei's Lab at CIW Geophysical Lab
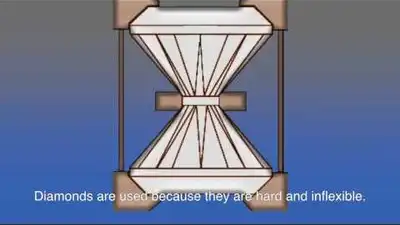
Diamond Anvil Cell