
Our Research
We utilize experimental and theoretical approaches to understand fundamental chemical processes and interactions and to create new and advanced technological and energy-related materials. We use the variable of pressure to synthesize new materials and use a range of spectroscopic techniques to interrogate their structures and properties.
We utilize theoretical approaches to predict and search for new compounds and syntheses routes for making stable compounds with desired properties at ambient conditions.
Top Questions
Generally speaking, materials under high pressures shrink in volume because the average space between atoms gets smaller. This new close-knit configuration allows different types of bonds to form between atoms, which can fundamentally change the properties of a material—like its electrical conductivity, thermal conductivity, viscosity, melting, and reaction kinetics.
Under ambient conditions, silicon and carbon atoms are arranged in a diamond-like crystal structure (left image). Above 1 million atmospheres (100 GPa), silicon carbide (SiC) is transformed to a rock-salt structure, in which the atoms are squeezed much closer together (right). These are two different phases of silicon carbide. Image courtesy Carnegie Institution for Science/Sally June Tracy.
Pressure generally changes a material’s properties on a smooth continuum—until it doesn’t!
At specific pressures, some materials’ properties change dramatically. These reproducible material boundaries designate phase changes in which the entire structure of the material suddenly and reliably rearranges. For example, molecular solids, like water ice, go through several phases of structural transition as pressure increases.
Scientists like those at EPL investigate the changing properties of materials under pressure. They constantly seek to expand their understanding of materials to apply that knowledge to models of planetary interiors and seek out new materials with properties that can help us expand our current technologies.
In a recent Neighborhood Lecture, someone in the audience asked Carnegie’s Tim Strobel what the top global challenges materials science can help solve.
“Clean water, renewable energy, world peace—you name it, and materials science can help solve it,” said Strobel. “Just look around where you’re sitting right now; maybe you’re at your desk looking at your computer screen. These are all materials with particular properties. The world we live in is possible because of materials science and novel materials research”.
In addition to allowing scientists to directly observe minerals behaving as they would in the center of the planet, EPL researchers like Strobel harness these high-pressure tools to create brand new materials that usually* only form under very specific high-temperature and pressure conditions. We now use computational chemistry to predict materials and then work in the lab to synthesize them and study their properties.
Things like next-generation superconductors, photovoltaics, and insulators are all on the table when pressure is involved.
We cannot take direct samples from the deepest interior of our planet. The Earth’s core is about 2,900 km below the surface. Meanwhile, the furthest we’ve managed to drill is only about 12 km, which doesn’t even get us past the crust and extend into the mantle. Sometimes we can find natural samples of high-pressure materials at meteorite impact sites or trapped inside of a diamond, but normally the best we can do is take samples of the materials we think are inside our planet and test them in the lab.
We perform this foundational research using high-pressure devices such as diamond anvil cells and large-volume presses such as the piston cylinder. We load precursor materials into these devices and then subject them to high pressures and temperatures to push the material to the limit. Scientists create detailed logs of how its mineralogy and properties change.
We can then use what we find to inform the physics and chemistry in computational models of planetary interiors—enhancing our understanding of how our planet developed and changed through time.
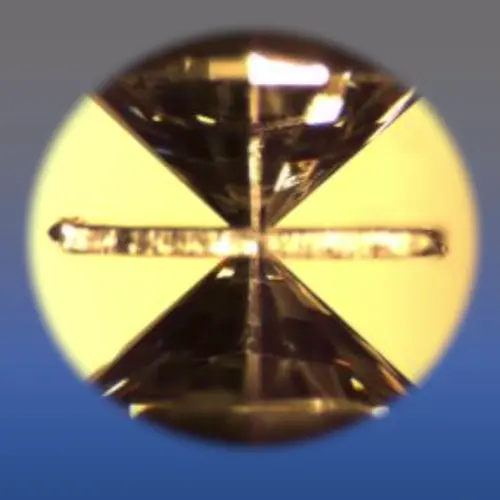
Tools of the Trade
High-pressure experiments are performed using piston cylinder and multi-anvil presses, laser-heated diamond anvil cells, and dynamic compression facilities. We analyze and characterize experimental samples using our wide-ranging in-house capabilities including SEM, EPMA, FIB, NMR, Raman, FTIR, PPMS, and X-ray diffraction.
We use national and international synchrotron facilities to probe samples in situ at micron and sub-micron scales. Theoretical approaches utilize high-performance computing systems both on and off-campus.
All instrumention