Overview
Timothy Strobel subjects materials to high pressures to understand chemical processes and interactions and to create new, advanced energy-related materials.
For instance, silicon is the second most abundant element in the Earth’s crust and a mainstay of the electronics industry. But normal silicon is not optimal for solar energy. In its conventional crystalline form, silicon is relatively inefficient at absorbing the wavelengths most prevalent in sunlight. Strobel made a discovery that may turn things around. Using the high-pressure techniques pioneered at Carnegie, he created a novel form of silicon with its atoms arranged in a cage-like structure. Unlike normal silicon, this new form has near-ideal properties for absorbing sunlight and could potentially usher in an entirely new generation of solar cells.
Understanding the chemical reactions that can create tiny molecular cages that hold other “guest” molecules—structures called clathrates—is key to creating a new generation of electronic devices and possible energy materials. Strobel and his team were the first to use high-pressure synthesis to create a stable clathrate of sodium (Na) and silicon (Si)—the least understood system of the so-called group 14 clathrates. Strobel also created a new clathrate of hydrogen sulfide (H2S) and molecular hydrogen (H2). Both findings open the door for major advances in materials science.
In addition to these experiments, Strobel and his team calculated how the materials would behave. Calculations and experiments revealed that sodium clathrates are thermodynamically stable at high pressure. The consistency suggests that scientists can use theoretical calculations to predict new synthesis routes for other compounds.
In other work, a team including Strobel reported the synthesis of an ionic semiconductor —Mg2C—that is fully recoverable to ambient conditions. Semiconductors are substances with electrical conductivity between insulators and metals used in electronic circuits.
Research
For any given pressure, temperature and composition, we can now predict and synthesize ground-state materials—those that lie on the convex hull– with remarkable success. However, numerous polytypes are predicted to exist above the convex hull, sometimes within only a few kJ/mol. In many cases, there are hundreds or thousands of “energetically plausible” and dynamically stable structures. Given the vast number of potential metastable structures compared with the singular ground state, it is likely that the material with the “best” property possible for a particular application is not the ground state. To what extent are metastable structures accessible? What criteria define accessibility and are there fundamental limits on synthesizability? Are there rational design principles and experimental methods to access these materials?
Of all physical variables, pressure possesses one of the greatest ranges—over sixty orders of magnitude. Pressure is thus a phenomenal tool to characterize fundamental interactions/processes and to gain access to new materials. As pressure is increased, the free energy of the system also increases through contributions to the pV term. At pressures approaching one million times atmospheric pressure, this contribution becomes comparable to the energy of a covalent bond and remarkable atomic arrangements become stabilized.
While the true energy-configurational landscape exists in high-dimensional (3N) space, it can be visualized in three dimensions by reducing the overall dimensionality. The global energy minimum represents the thermodynamic ground state, but many higher-energy local minima may be stabilized by the system's inability to overcome kinetic barriers. Take carbon for example. At low pressure, the lowest-energy structure is graphite, while diamond is the lowest-energy structure at high pressure. Once formed under high-pressure, high-temperature conditions, diamonds may be metastably recovered to ambient conditions, with robust kinetic persistence. Many new materials become thermodynamically stabilized under high-pressure conditions and can be recovered to ambient conditions.
New oxides, nitrides, and carbides –virtually every class of compounds– are waiting to be discovered.
In the past 15 years, we have seen tremendous advancements in computational algorithms that allow for accurate prediction of ground-state crystal structures given only information regarding chemical composition. For the case of structures on the convex hull, computational predictions are now remarkably successful. But what about the structures above the convex hull? Can computational prediction help guide actual synthesis pathways?
A long-term research goal is to predict synthesis pathways in order to help produce the most challenging metastable materials in the laboratory. What is the best starting point and processing pathway to obtain the exceptional materials that lie only slightly above the convex hull?
Many high-pressure materials that are recovered to ambient conditions were formed under conditions of thermodynamic stability. But this need not be the case, and entirely new metastable structures can be accessed by starting from metastable, high-energy states that can relax into local energy minima. One approach is to start with high-energy molecular or extended precursors. Once pressurized, kinetically controlled reaction pathways may become favorable, and the system need not fully relax to the global energy minimum. High-energy molecular precursors may also exhibit favorable topology such that single-crystal transitions to extended states are possible. New intermediate structures between well-known global minima (e.g., states that exist between graphite and diamond) may be obtained by starting from higher-energy extended structures (e.g., glassy carbon).
Recovered high-pressure materials contain stored pV energy, which can make them fascinating candidates for precursor states. These high-pressure precursors (HPPs) are often times metastable by ~30 kJ/mol and contain bonding arrangements and topologies that cannot be easily obtained by other methods. Once a material is synthesized at high pressure (it could be thermodynamically stable or metastable), it is recovered to ambient conditions (in which case it will definitely be metastable). This recovered material now serves as a high-energy precursor for additional chemical and/or physical manipulation. Due to the unique position of the HPP on the energy/configuration landscape, entirely new transformation pathways become possible.
While the high-pressure behavior of most pure molecules is established under high-pressure conditions, this is not true for mixtures and significant knowledge gaps remain, even for binary systems. At only a few kbar above atmospheric pressure, entirely new molecular compounds are found in simple systems like H2 and H2O. Many of these materials are dense with molecular hydrogen and could serve as useful energy storage materials. These types of compounds may also be important to planetary science with abundance on certain planetary bodies.
Fundamental interactions in molecular systems are often very interesting. Inelastic scattering methods, such as neutron and light scattering techniques, are extremely useful tools to probe this interaction in situ.
Laboratory Instrumentation
Strobel's lab has a wide range of diamond anvil cells available, with varying degrees of optical access and temperature control options for different applications. For example, low temperatures are achieved within a cryostat (Cryoindustries DAC He cryostat) and high temperatures are achieved using resistive or laser heating (300W IPG IR fiber laser).
Two gas loading facilities are available to load gases within the DAC to achieve quasihydrostatic conditions (or when gas is part of your sample of interest!).
Turn the drive screws of the DAC to generate pressure, or use the Druck Pace5000 pressure controllers, which allow for precise application (or removal) of pressure for membrane (or double membrane) DAC experiments.
The LABstar glovebox allows for experiments to be conducted and manipulated under an inert Ar atmosphere. The glove box is equipped with two antechambers, one small and one large, to allow for more efficient cycling. The box is equipped with a single column inert gas purification system that maintains O2 and H20 levels below 1 ppm. The interior of the box is equipped with a diamond anvil cell loading stage (camera, monitor, light sources), a balance accurate to 0.001 g, pellet press, and a vacuum sealing system. Available chemicals are maintained in an inventory list stored next to the glovebox.
A variety of furnaces and ovens are available for synthesis. The Mellen microtherm furnace is an industrial box furnace setup for solid-state synthesis. The furnace can maintain temperatures up to 1800 °C and heats a volume of 6”x6”x6”. The oven is controlled by an Omega CN7800 controller and can run a variety of temperature profiles including slow heating and cooling. The Mellen split tube furnace operates at temperatures up to 1250ºC in air. The standard orientation of the furnace is horizontal, however, a vertical stand or a bench stand is available. The furnace has fast heat-up rates (30ºC per minute standard) and an integrated dual-display controller, making it easy to use. Tubes are easily attached to the turbo pump for dynamic pumping experiments. The Thermolyne small muffle furnace is the ideal choice for quick runs up to 1100ºC. The digital setpoint controller allows for a variety of custom heating profiles. The isotemp vacuum oven is an ideal setup for general vacuum drying and heating applications. The oven has a depth of 18” and a capacity of 1.5 cu. Ft. The oven can maintain temperatures up to 280 °C and can be monitored with the built-in LED controller. The controller can be set up for programs as long as seven days and can cycle on and off up to eight times per day.
The vacuum sealing line is used to isolate solid-state experiments under vacuum in glass tubing. The line is setup to receive various sizes of glass tubing, evacuate them, and seal the tubing with a hydrogen/oxygen torch. The system can maintain vacuum to at least 10-3 Torr during sealing.
The Fritsch pulverisette is suited for loss-free grinding to 100 nm of hard, medium-hard, and brittle materials. Grinding can be performed dry, in suspension, or in inert gas. The system maintains main disk rotational speeds of 100-1100 rpm and features a SelfLOCK sample chamber for absolutely safe operation. The system can run simultaneously on two samples with a capacity of 30 ml each.
Strobel maintains three X-ray diffractometers for in-house structural studies
1) Bruker D8 Discover with Cu microfocus source. This multi-function X-ray diffractometer allows microanalysis with interchangeable collimators from 2mm-0.1mm. X,Y,Z translation is coupled with tilt and rotation for micro-positioning. Reflection, in-plane grazing incidence diffraction, small-angle X-ray scattering, and transmission measurement geometries are accommodated.
Users can choose the 140 mm diameter Vantec500 area detector or the high-resolution LynxeyeXE 1-D detector for the desired speed/resolution.
2) Bruker D2 Phaser (Cu) with Lynxeye 1-D detector for general powder diffraction. This powder X-ray diffractometer is quick and easy to use for obtaining high quality data in reflection geometry. An integrated flat screen allows real-time data monitoring.
3) The "Ingaku" is an old Rigaku D/max hutch upgraded with a high-brilliance Incoatec Ag microfocus source. The system is built around a robust Huber 4-circle goniometer with scintillation detector for high-quality single crystal studies.
The 250 ton Paris-Edinburgh Press (VX3) is equipped with a Teledyne Isco syringe pump (30 ml capacity, 30,000 PSI) and Keysight power supply unit (9 V-220 A) and is used for recovering cubic millimeters of samples from high-pressure and high-temperature (HP-HT) synthesis experiments. The ‘conoidal’ anvils, pyrophyllite gaskets and cylindrical graphite heaters are currently used for high-temperature experiments. The typical pressure and temperatures routinely used are 6-9 GPa and 1600K.
This setup also has the capability to perform resistivity measurements under pressure using Keithley digital multi-meter. High-pressure experiments to 25 GPa are possible using a metallic gasket with sintered diamond anvils. In collaboration with Yingwei Fei, we also perform materials synthesis experiments using the large-volume multianvil press lab, generating pressures up to 25 GPa and temperatures up to 2300 K. In collaboration with Reini Boehler, we are working to develop novel large-volume synthesis capabilities.
Two 3D printing stations for rapid prototyping and for true 3D visualization of the crystal structures.
Strobel use a variety of spectroscopic and optical characterization methods.
The high-resolution Raman spectrometer is built around a Princeton Instruments SP2750 spectrograph with PyLoN CCD. The SP2750 spectrograph has a focal length of 750 mm, aperture ratio of f/9.7, PMT resolution of 0.03 nm, CCD resolution of 0.06 nm and Linear dispersion of 1.03 nm/mm. The PyLoN CCD camera is liquid-nitrogen-cooled down to -120°C. The green single-frequency torus laser excitation wavelength is 532nm, with power of 150 mW. Three gratings of 300 g/mm, 1800 g/mm, and 2400 g/mm are available. LightField software is used to control the camera and spectrograph.
The Bruker Vertex with Hyperion microscope is used to collect Fourier transform spectra from the mid-IR to the UV.
The Perkin-Elmer Lambda950 spectrophotometer is equipped with a 150mm InGaAs integrating sphere and PMT/InGaAs detector which work in the operating range 175-2500nm. The instrument is routinely used to measure absorbance, transmittance/reflectance of powders, pellets, recovered DAC gaskets and thin films. The integrating sphere expands the measurement capability of this instrument to include the total and diffuse reflectance of both specular and diffuse samples.
CV
- Ph.D., Chemical Engineering, August 2008
Dissertation: “On Some Clathrates of Hydrogen”
Advisors: Professor E. Dendy Sloan, Professor Carolyn A. Koh
Minor subject: Chemistry - B.S., Chemical Engineering, May 2004
- American Chemical Society
- American Physical Society
- Materials Research Society
- Carnegie Institution for Science, Earth and Planets Laboratory, Washington, DC, USA
- Staff Scientist September 2011 to Present
- Associate Director - EFree, DOE EFRC August 2014 to July 2019
- Research Scientist October 2010 to August 2012
- Carnegie Fellowship September 2008 to September 2010
- Colorado School of Mines Chemical Engineering Department, Golden, CO, USA
- Research Assistant October 2003 to August 2008
Photos and Media
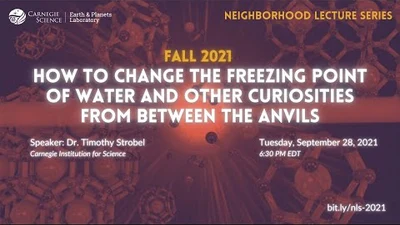
How to change the freezing point of water and other curiosities from between the anvils.
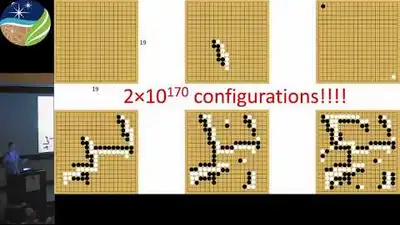
Geophysical Laboratory Neighborhood Lecture Series - Tim Strobel