Overview
Earth is the only planet we know of that has maintained a strong magnetic field, plate tectonics, surface liquid water, and life over billions of years.
What is it about Earth’s interior that has allowed these complex phenomena to occur? How do they work? Are they connected in any way?
Driscoll uses first-principles numerical magnetohydrodynamic simulations to investigate dynamo behavior over a range of parameters and timescales. In addition, he develops 1D models of heat and mass transfer within the interior. The evolution of the deep interior is manifested in paleomagnetic and tectonic observations that require an understanding of the long-term coupling of the mantle and core.
In particular, he is focused on how the evolution of the geodynamo over the last 500 million years is related to convective cycles in the mantle, the growth of the solid inner core, and changes in rotation. He investigates the process of magnetic polarity reversals by comparing numerical dynamo simulations to geomagnetic observations and pushes towards more realistic Earth-like dynamo simulations.
He is also investigating the dynamics of rocky exoplanets, in particular through coupling internal and orbital evolution models to make predictions for their detectability and habitability.
Research
The geodynamo is the process by which Earth generates its magnetic field by convection in the liquid iron-rich outer core. Evidence supporting the existence of the ancient geodynamo extends back to at least 3.5 billion years ago—possibly as far back as 4.2 billion years, nearly the age of the Earth itself.
How has Earth maintained a dynamo-generated magnetic field for so long? No one knows for sure, but the answer is intertwined with how Earth has maintained a habitable surface for so long.
The geodynamo that creates the magnetic field is a critical component of Earth's prolonged habitability. It shields the surface of our planet from high-energy particles that are harmful to life. It might even be important for protecting the atmosphere from erosion by solar wind. Understanding what drives the geodynamo and how it may have evolved over Earth's history is central to understanding the evolution of life, the atmosphere, and what makes planets habitable in general.
Earth is the only planet known to have maintained an internally generated magnetic field in its core over most, if not all, of its history. The presence of a core-generated planetary magnetic field is commonplace in the Solar System, with nearly every planet either maintaining a present-day core dynamo (Earth, Mercury, Jupiter, Saturn, Uranus, Neptune) or showing evidence of one in the past (Mars). Although there is presently no evidence for a dynamo in Venus, it could have maintained one in the past. Even satellites are capable of dynamo action: lunar rocks show evidence of a now-extinct dynamo in the Moon, and Ganymede, the largest satellite of Jupiter, maintains a dynamo today.
There are three general requirements to maintaining a planetary magnetic field:
- The presence of a liquid that is electrically conductive—like Earth’s iron-rich liquid outer core
- Planetary rotation
- Fluid motion—often generated by the convection of the fluid
Factors (1) and (2) are common but the presence of convection is less of a guarantee.
To drive convection in the core there must be a continuous source of buoyancy to keep the fluid moving. In the modern-day core, this is thought to be predominantly supplied by the release of light elements at the base of the outer core, where the iron-rich liquid solidifies onto the solid inner core. During the solidification, light elements—such as oxygen, silicon, or sulfur—are rejected from the solid and accumulate in the liquid, where they rise and drive convective currents. However, Earth’s inner core, and the solidification process, is likely only about a billion years old based on the cooling rate of the planet’s interior, which is significantly younger than the geodynamo.
Peter Driscoll is currently working on modeling the thermal evolution of the Earth to better constrain the timing of inner core solidification and the cooling rate of the interior, which is critical to understanding what was driving the geodynamo in the deep past.
He is also working with Computational Scientist Cian Wilson on first principles 3D dynamo models that generate magnetic fields from rotating convection in a spherical shell. In particular, they are testing how the thermal and chemical buoyancy fields interact and are coupled to each other as the core cools. Ultimately their goal is to better quantify what kinds of magnetic fields are generated from different driving forces and apply that knowledge to better understand the Earth’s thermal evolution from the paleomagnetic record.
- Chair, Computational Infrastructure for Geodynamics, Dynamo Working Group 2019-present
- Associate Editor, Frontiers in Geophysics, 2018-present
- Carnegie Venture Grant 2019, 2017
- Yale University Bateman Fellowship, 2010-12
- NASA Ames Honor Award 2002
One possibility is that prior to inner core solidification the liquid core was cooling fast enough to drive thermal convection, where the heat being removed exceeded the amount that can be transported conductively, that is without fluid motion. The issue with thermal convection is that iron is a very good conductor of heat, so the core would have had to be cooling rapidly to drive thermal convection. Moreover, the cooling rate would have had to be high for billions of years while not so fast as to freeze the entire core!
Another possibility is that one or more of the light elements in the core could be exsolving (or precipitating) out of the core as it cools. In this scenario, a light element phase (such as SiO2) that was once stable in the iron alloy when the core was hotter becomes unstable as the core cools, and separates from the iron alloy at the top of the core. Below where the two liquids separate an iron-rich liquid residue will form and drip down into the liquid core, driving convective currents.
- First to predict that Earth’s inner core nucleated around 600 million years ago and that the lack of paleomagnetic data around that time is due to the magnetic field being abnormal (non-dipolar) because it came close to shutting down (see Driscoll, GRL, 2016)
- First to predict Earth-like exoplanet magnetic field radio emissions (see Driscoll & Olson, ICARUS, 2010)
- First to systematically analyze how magnetic reversal frequency evolves with core heat flow and rotation rate (see Driscoll & Olson, 2009a, b ; Driscoll & Olson, GRL, 2010)
- First to identify geodynamo conductivity limits, which places theoretical limits on the magnetic induction rate of a terrestrial dynamo (see Driscoll & Du, 2019)
- First to quantify how paleomagnetic bias depends on magnetic reversal rate (see Driscoll & Wilson, 2018)
CV
- Ph.D., Earth & Planetary Sciences, Johns Hopkins University, Baltimore, MD, 2010
- M.A., Earth & Planetary Sciences, Johns Hopkins University, Baltimore, MD, 2008
- M.S., Physics & Astronomy, San Francisco State University, San Francisco, CA, 2006
- B.S., Physics & Astronomy, Dickinson College, Carlisle, PA, 2003
- Staff Scientist, Earth & Planets Lab, Carnegie Institution for Science, 2015-present
- Postdoctoral Fellow, NASA Virtual Planetary Laboratory, University of Washington, 2013-2015
- NSF FESD Postdoctoral Researcher, Yale University, 2012-2013
- Bateman Postdoctoral Associate, Yale University, 2010-2012
- NSF Graduate Research Associate, Johns Hopkins University, 2006-2010
- Graduate Teaching Associate, Johns Hopkins University, Spring 2008
- NASA/NSF Graduate Research Associate, California Planet Search, San Francisco State U., 2003-2006
- Graduate Teaching Associate, San Francisco State University, 2003-2006
- Education Associates Internship, NASA Ames Research Center, Summer 2002
- Teaching Assistant & Laboratory Consultant, Dickinson College, 2001-2002
- NSF Summer Internship, Scitefair International, Inc., Philadelphia, PA, Summers 2000-2001
- Planetary Habitability, Guest Lecturer, University of Washington, Fall 2013, 2017
- Early Earth Seminar, CoOrganizer, Yale University, Spring 2013
- Life in the Universe, Guest Lecturer, Yale University, Fall 2011
- Habitable Planets Seminar, Co-Organizer, Yale University, 2010-2011
- Natural Catastrophes, Assistant, Johns Hopkins University, Spring 2008
- Physical Sciences, Guest Lecturer, Johns Hopkins School of Education, Spring 2007
- Introductory Astronomy Lab, Instructor, San Francisco State University, 2004-2006
- Introductory Physics Lab, Instructor, San Francisco State University, Fall 2003
- Workshop Physics, Assistant, Dickinson College, 2002-2003
- American Geophysical Union (SEDI, GP) 2007-20
- Computational Infrastructure for Geodynamics
- Dynamo Working Group (Chair)
- Studies of Earth Deep Interior 2009-20
- American Astronomical Society
- Keck Institute for Space Studies
- Carnegie Venture Grant 2019, 2017
- NASA Astrobiology Institute Fellow 2013-15
- Yale University Bateman Fellowship, 2010-12
- NSF Graduate Research Assistant 2008-10
- NASA Ames Honor Award, Summer 2002
- NASA Ames Education Associate, Summer 2002
- SciTech Scholarship, 2002-03
- Goldie Kunkle Beal Scholarship, 2002-03
- Dickinson College Grant, 1999-2003
- UCLA, Earth, Planetary, & Space Sciences (remote), May 2021
- American Geophysical Union, Various Locations, December 2007, 2008, 2009, 2010, 2011, 2012, 2013, 2014, 2015, 2016, 2017, 2018, 2019, 2020
- COMPRES Keynote Lecture Series (remote), August 2020
- Princeton University, Dept. Geosciences, September 2012, November 2019 ‡
- Exoplanetary Worlds Workshop, Carnegie Science, October 2019
- United States Naval Observatory, Washington DC, May 2019
- Neighborhood Lecture, Department of Terrestrial Magnetism, November 2018
- Johns Hopkins University, Earth & Planetary Sciences, Bromery Lecture, Baltimore, MD, November 2015
- 50th Anniversary Symposium, Keynote Lecture, June 2018
- Geological Society of Washington, Cosmos Club, Washington, D.C., March 2018
- Rutgers University, Earth & Planetary Sciences, New Brunswick, NJ February 2018
- NASA Goddard, Greenbelt, MD, March 2017
- University of Maryland, Department of Geology, MD, October 2016
- Computational Infrastructure for Geodynamics, Keynote Lecture, UC Davis, CA, June 2016
- George Mason University, Earth Sciences, Fairfax, VA, April 2016
- University of Pennsylvania, Physics & Astronomy, PA, March 2016
- University of Cambridge, Melt in the Mantle Workshop, Isaac Newton Institute, UK, February 2016
- Carnegie Institution for Science, Carnegie Origins Workshop, Washington DC, October 2015
- American Astronomical Society, Various Locations, January 2005 *; January 2006 *; 2010 *; 2015
- Division for Planetary Sciences Conference, Tucson, AZ, November 2014
- Carnegie Institution for Science, Dept. Terrestrial Magnetism, Washington, D.C., June 2014
- Deep Carbon Modeling Workshop June 2014
- Exoplanets, Biosignatures, and Instruments Conference, Tucson, AZ, March 2014
- California Institute of Technology, Keck Institute for Space Science, Pasadena, CA, February 2014
- University of Washington, Astrobiology, October 2013
- International Association of Geomagnetism and Aeronomy, Merida, Mexico, 2013
- Tokyo Institute of Technology, Earth Life Science Institute Symposium, Tokyo, Japan, March 2013
- Yale University, Geology & Geophysics Colloquium, New Haven, CT, February 2013
- Harvard University, Earth and Planetary Science Seminar, Cambridge, MA, February 2013
Media and Recorded Talks
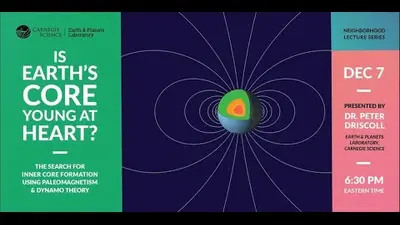
Is Earth’s core young at heart? Search for inner core formation using paleomagnetism & dynamo theory
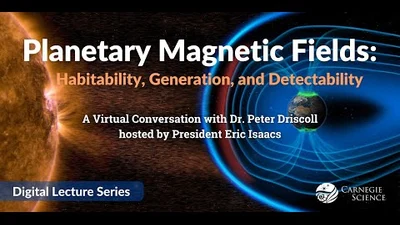
Planetary Magnetic Fields: Habitability, Generation, and Detectability
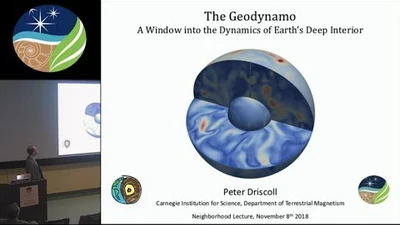
The Geodynamo: A Unique Window Into the Dynamics of Earth's Deep Interior
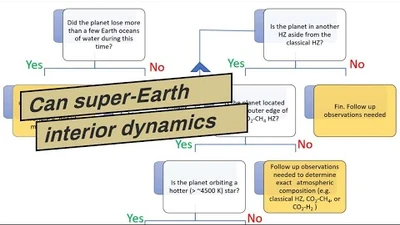
Can super-Earth interior dynamics set the table for habitability?