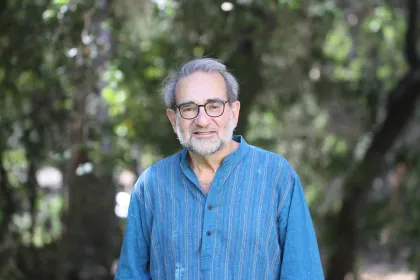
Arthur Grossman didn’t set out to develop interdisciplinary expertise across plant sciences. It emerged as a result of his natural curiosity, and the freedom, flexibility, and innovation encouraged by Carnegie Science’s leadership.
Grossman’s research has ranged from plant biology to marine biology and from microbiology to genomics. Over the course of four decades at Carnegie Science, he has advanced our understanding of photosynthetic organisms from a variety of perspectives, often with exciting possible biotechnological applications.
Earlier this year he was selected as one of 120 new U.S. members of the National Academy of Sciences in recognition of distinguished and continuing achievements in original research—one of the scientific community’s highest honors.
“When working on a project, you often see paths that extend it into new and exciting directions,” Grossman explains.
Grossman credits his freedom to pursue new questions as they arise to the organization’s model for research, which empowers top scientific minds to explore questions at the frontier of science.
“In my four decades at Carnegie Science’s Palo Alto campus, there has never been a sense of staying put,” he adds. “We are always dedicated to the idea of breaking boundaries. Throughout my career, we have consistently quested to open up new areas of research and explore novel scientific ideas—even those associated with difficult challenges. Looking ahead, I am hopeful that the organization will maintain both the financial support and intellectual foundation that enables scientists to use their imagination and creativity in pursuit of understanding the complexities of life.”
Grossman notes that his approach is also oriented toward setting students and postdocs up for future success by encouraging them to use the information and ideas generated during their tenure in the lab to help build their own exciting research programs. Indeed, many of Grossman’s former mentees have launched successful academic and industry careers and continue to collaborate with him as they advance.
Grossman’s drive to keep moving forward is combined with a fearlessness about adopting new technologies as they emerge. When he started his Staff Scientist position at Carnegie in 1982, he was working on photosynthetic microbes called cyanobacteria and the green alga Chlamydomonas, trying to understand how these organisms perceive and respond to their environment—especially to the color, quality, and intensity of light, as well as the availability of carbon dioxide and soil nutrients.
These interests led to exciting collaborations with colleagues, including Joe Berry and the late Winslow Briggs and Olle Björkman, while also extending his reach to vascular plants, red algae, and dinoflagellates—single celled marine and freshwater plankton.
It was through his work on Chlamydomonas that Grossman became an early adopter of genome sequencing for exploring biological processes in photosynthetic organisms.
“I realized at some point that Chlamydomonas research—and algal research more broadly—would only push forward—make significant progress—if the genomes were characterized,” Grossman says. “At that point, genome projects were relatively new and the process of designing one was much more technological than biological, which is why some colleagues discouraged me from undertaking it. But I decided that it needed to be done and I was able to do so because of the freedom that Carnegie Science afforded me.”
After Grossman and his collaborators obtained and characterized the Chlamydomonas genome, he went on to serve on the steering committee for genome sequencing of the red algae Porphyra umbilicalis. His lab has continued to use this and other cutting-edge ‘omics techniques to reveal molecular mechanisms of photosynthesis and the evolution of the cellular organelle, called the chloroplast, where this crucial biochemical process occurs.
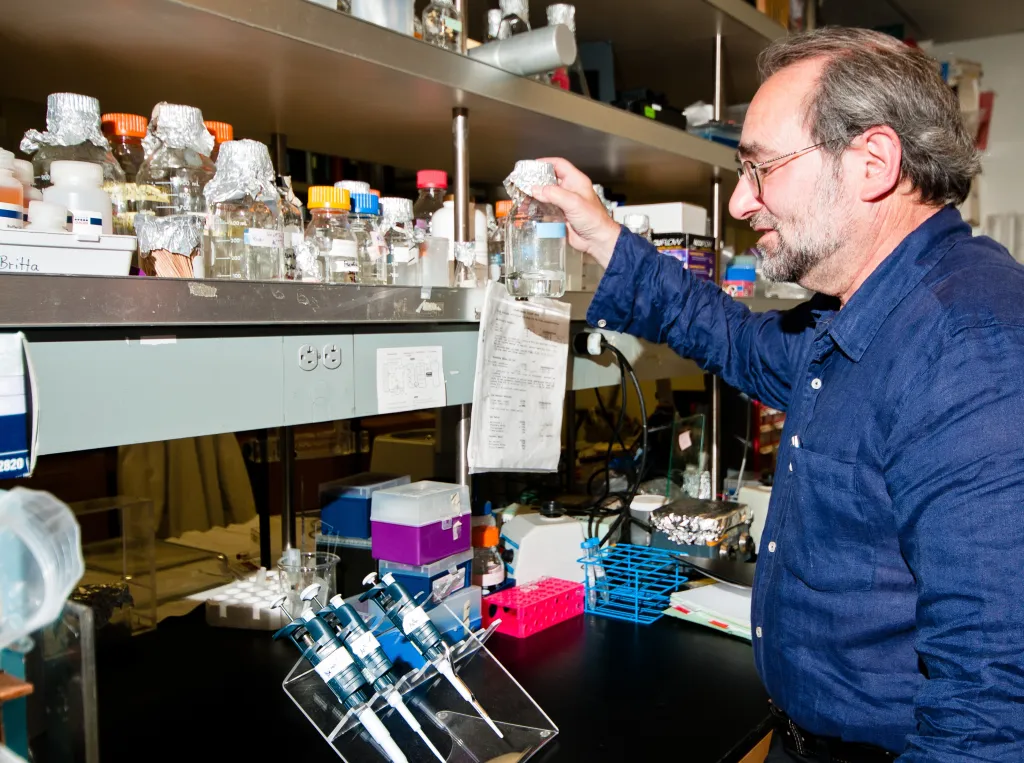
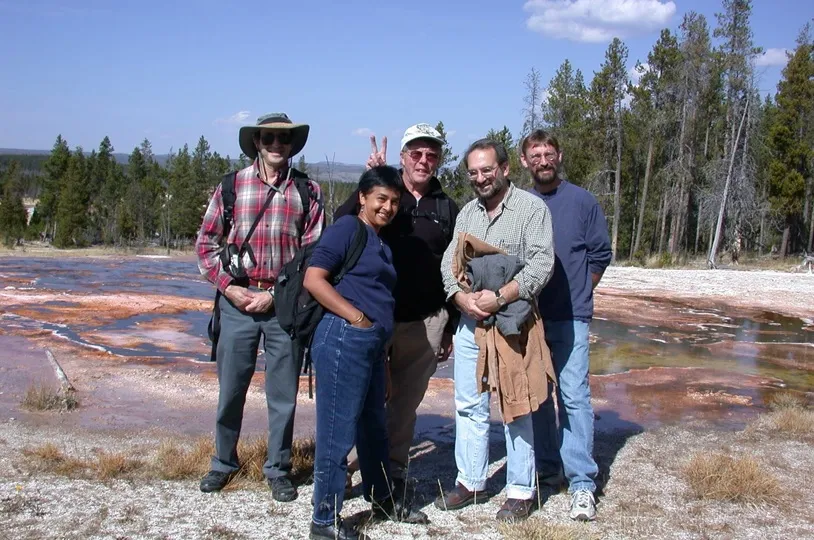
Arthur Grossman joined a fieldwork team led by Carnegie Science's Devaki Bhaya at Yellowstone National Park.
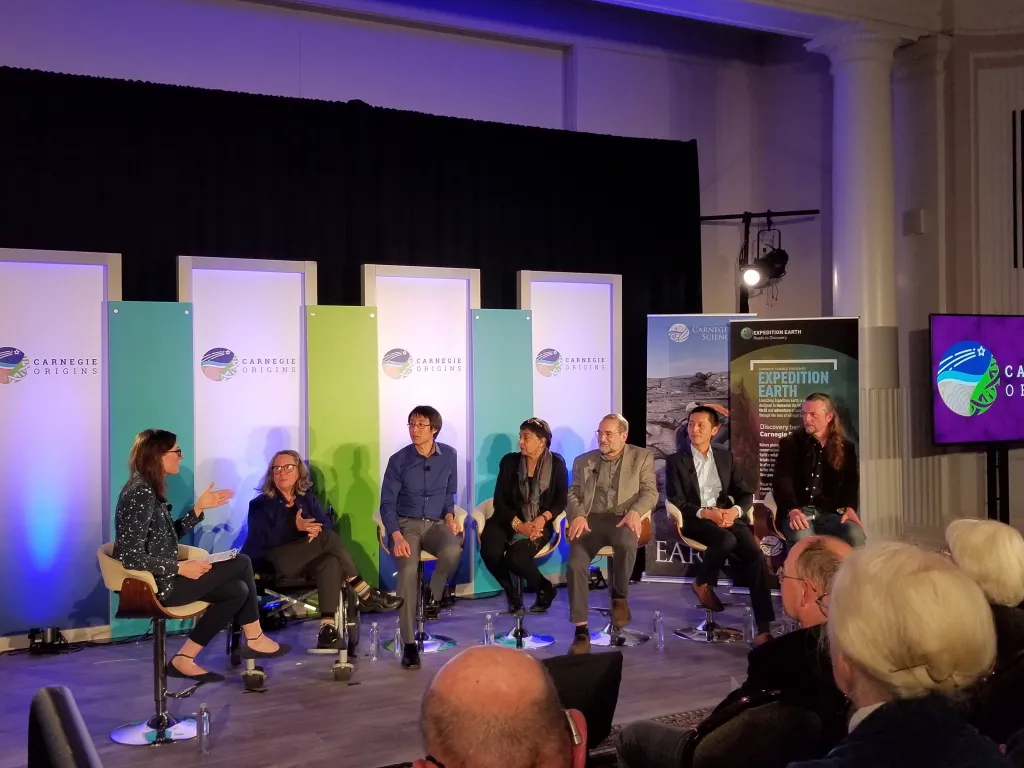
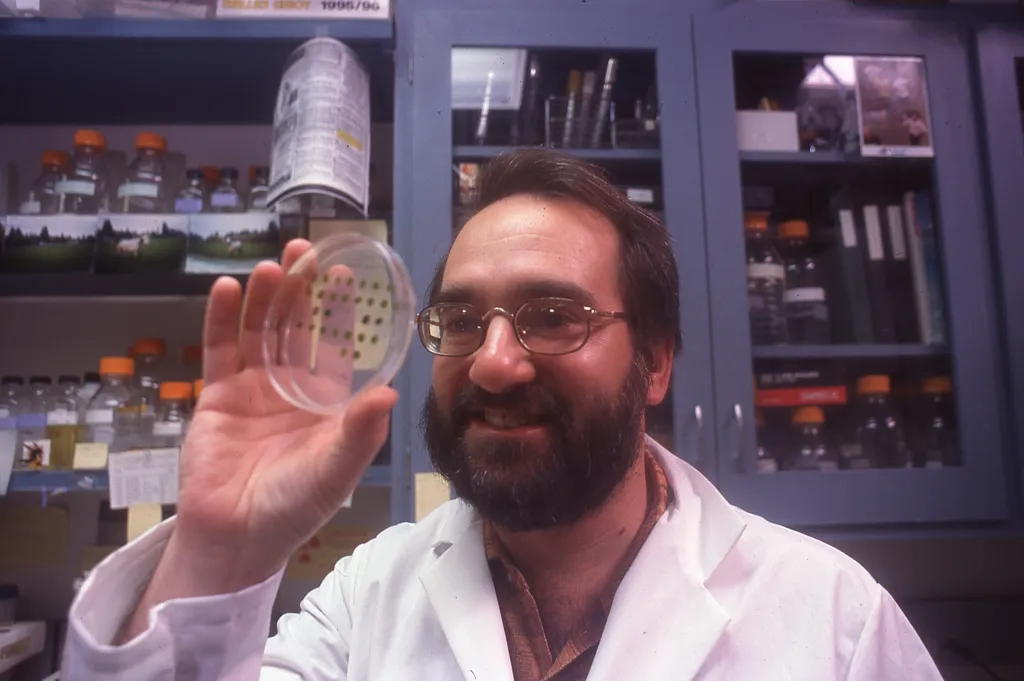
Arthur Grossman examines a petri dish in his lab in 1997.
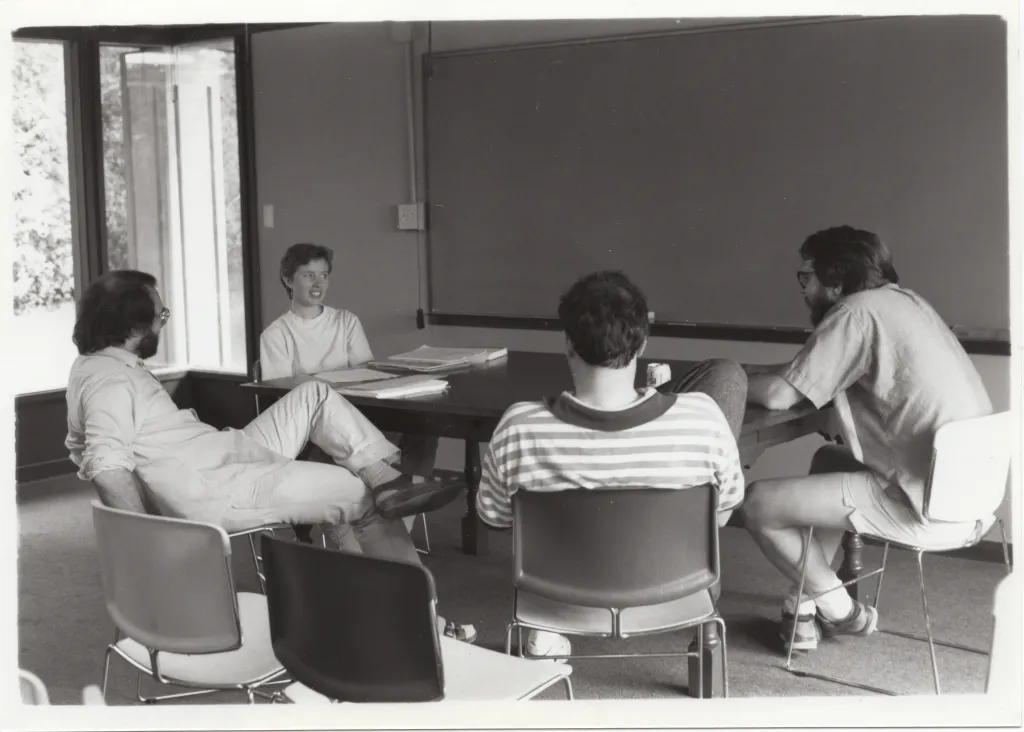
Arthur Grossman and lab members sit around a table to discuss their research in 1985.
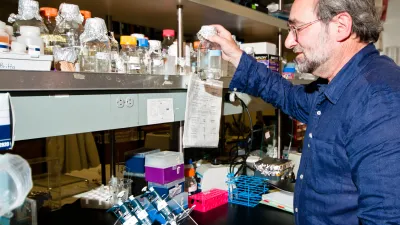
Arthur Grossman in the lab
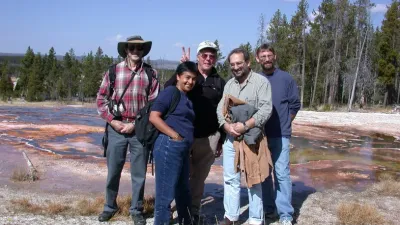
Hot spring fieldwork
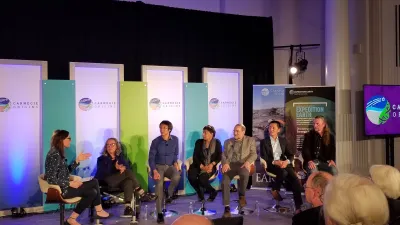
Carnegie Science Origins series
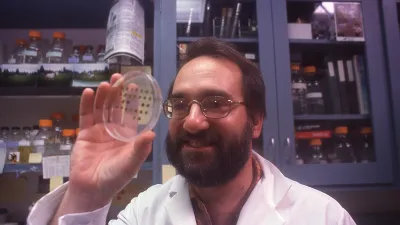
Grossman with petri dish
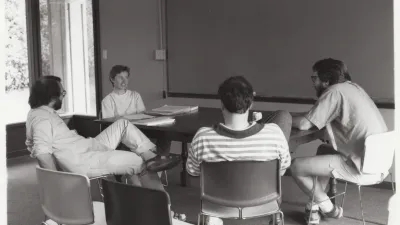
Grossman lab 1985
Omics is the collective effort to identify, characterize, and quantify groups of biomolecules within a cell or in a community and to understand their contributions to the complex physiology of the organism or to dynamic group interactions. It represents the intersection of a variety of specialties, including genomics, transcriptomics, proteomics, and metabolomics. Looking ahead, Grossman says, the ability to unveil connections in the massive quantities of data that ‘omics research yields will enable big-picture breakthroughs in biology.
“Many people are doing ‘omics research—pushing buttons, identifying genes, clustering genes, finding analogous genes across organisms—but, in my opinion, to confidently put this information into a biological context you, we need to perform experiments that validate and expand upon the many hypotheses that arise from ‘omics analyses,” Grossman explains. “There’s a wealth of information that you could mine for years and years, but to develop knowledge that could create a powerful new understanding of living systems, you need to integrate genome-wide analyses with the biochemistry, physiology, and ecology of the cells.”
Grossman’s commitment to applying new techniques to long-standing biological questions also extends beyond algae to incorporate corals and other marine organisms. He was a forerunner in adopting the tools of molecular biology for exploring marine conservation—a very hot research area—investigating mechanisms of coral symbiosis and the causes of coral bleaching.
Coral, jellyfish, and sea anemones all have symbiotic relationships with similar species of photosynthetic algae. Because these algae are photosynthetic, they can convert the Sun’s energy into chemical energy in the form of sugars and fats. These nutrients are shared with the host animal, which in turn provides them with shelter. His work probes the basis of this mutually beneficial relationship.
This is part of another research horizon that Grossman says that is rapidly becoming a focus of many groups of life scientists—investigating the molecular underpinnings of interactions among organisms and between species within an ecosystem.
“For an organism to exist as it would in nature and to go through all the stages of its life cycle, it needs to be part of a community and interact with its neighbors,” Grossman elucidates. “However, there is so much about these kinds of relationships that we don’t understand because we mostly study model organisms in isolation from the other species that populate their communities in nature.”
These types of investigations into the molecular processes and dynamics underlying community relationships will require major investments and interdisciplinary teams, according to Grossman. Bringing diverse expertise together in this way will encourage researchers to consider different perspectives and probe existing questions from new angles, he adds, saying that “from an interdisciplinary point of view, the field is much more progressive now than it was when I first began.”
With this in mind, Grossman encourages early career scientists in his lab to communicate and be open to novel ideas and directions in choosing a career paths—which could include academics, science advocacy, technical writing, consulting and industry work—'being open and considering various options will likely result in a suite of exciting career opportunities.
Science is highly dynamic, he concludes, saying: “I think you have to be prepared for change, flexible in your approaches and seek out valuable collaborations that introduce you to new technologies, novel ideas, and the joys of commitment.”