Massive and gassy, Jupiter and Saturn are planets most people know. But perhaps what many people don't know is that similar gas giants are quite common in other planetary systems. Scientists are currently studying how these planets form, and why they are so different from rocky and gas-poor worlds like Earth. That mystery is one of toughest problems in planetary science.
The core accretion mechanism, one of the most widely accepted theories for gas giant formation, holds that large planets can pull in gas to form an atmosphere, eventually becoming huge gas worlds. In a new study based on this mechanism, DTM astrophysicist John Chambers found that gas giants in early stages of formation may have had oceans with steaming atmospheres before growing into gas-rich, Jupiter-like giants. The study is available as an early online release by The Astrophysical Journal.
Chambers used computer models to simulate formation. In this new Q&A, he tells us all about his "virtual" worlds, what his study suggests about gas giant formation, and how that can help us to better understand how planets form in and beyond our Solar System.
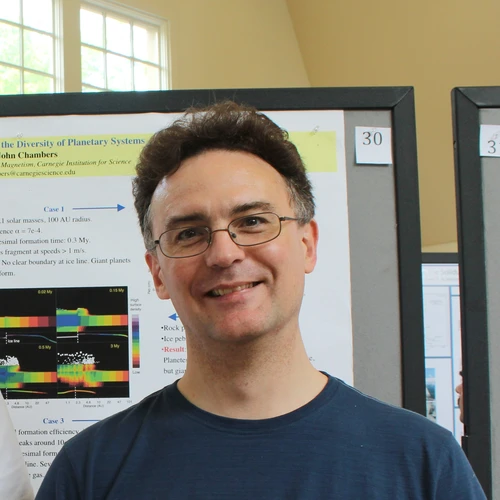
Astrophysicist John Chambers uses computer simulations to model the formation of planetary systems.
What did your model show in your most recently published study?
I found that planets somewhat smaller than the Moon are large enough to capture a thin atmosphere. This atmosphere grows thicker and hotter as the planet gets bigger. Once the planet is similar to Mars in size, it can form an ocean. At larger masses, the planet's ocean boils and the atmosphere becomes a dense mixture of steam and hydrogen and helium. When a planet reaches a few times the mass of Earth, the atmosphere will grow rapidly, faster than the solid part of the planet, eventually forming a gas giant planet like Jupiter.
I simulated the growth of a solid planet in a cloud of gas surrounding a young star. I calculated whether the planet would be able to capture an atmosphere, what this atmosphere would be made of, how heavy the atmosphere would be, and whether the planet would have an ocean. I specifically looked at planets that form in locations that are cold enough for water ice to be present. This ice together with rock provides the material to build a solid planet.
This sequence of events for a growing planet should be quite robust. However, the precise size of the planet at each stage is somewhat uncertain and depends on factors that we continue to study, such as the ice and rock mixture in the solid materials that form the planet and how fast the solid planet accumulates.
What kind of planets does your model see forming under these circumstances?
Many young stars are surrounded by flattened clouds of gas and dust, called protoplanetary disks, that seem to be the starting materials for planet formation. All protoplanetary disks are thought to be mainly composed of hydrogen and helium gas, since these are the most common materials in the universe. These are also the most abundant materials in the Sun and its largest planets, Jupiter and Saturn. Planets that grow massive enough to pull in huge amounts of gas from a protoplanetary disk should be mainly composed of these gases as a result. However, smaller planets could have a different composition. If a planet doesn't accrete too much hydrogen and helium gas, it may be dominated by materials that would be ices at low temperatures. These planets would resemble Uranus and Neptune, which have some hydrogen and helium, but are mostly composed of gases like steam and methane.
The formation of a gas giant
A planet acquires an atmosphere if gas is available and if the planet's gravity is strong enough to stop the atmosphere escaping. First, the planet is very small, and its gravity is too weak to hold on to an atmosphere. The gravity becomes stronger as the planet grows large enough to capture and retain some hydrogen and helium gas, continually sweeping up ice-rich pebbles. As these pebbles fall through the planet's atmosphere, a fraction of the ice evaporates, adding water vapor to the atmosphere. The remaining material in the pebbles falls to the surface, which is cold enough for the ice to remain solid. The outer atmosphere is very thin, whereas the lower atmosphere is thicker. This region may have clouds and weather. As the planet continues to grow, its atmosphere is denser and hotter. Infalling pebbles lose more of their ice as they fall through the atmosphere. In the lower atmosphere, any ice remaining in the falling pebbles melts, and the water falls to the surface to form an ocean. When the planet grows and its atmosphere is hotter, pebbles fall through the atmosphere, all their ice evaporates, and only the rocky portion of the pebbles reaches the solid planetary surface. Water can exist as a liquid at intermediate altitudes in the atmosphere, and melting ice will form rain. Low in the atmosphere, temperatures are too high for liquid water to exist, and water becomes steam that mixes with the hydrogen and helium gas. Adapted from "Steamworlds: Atmospheric Structure and Critical Mass of Planets Accreting Icy Pebbles."
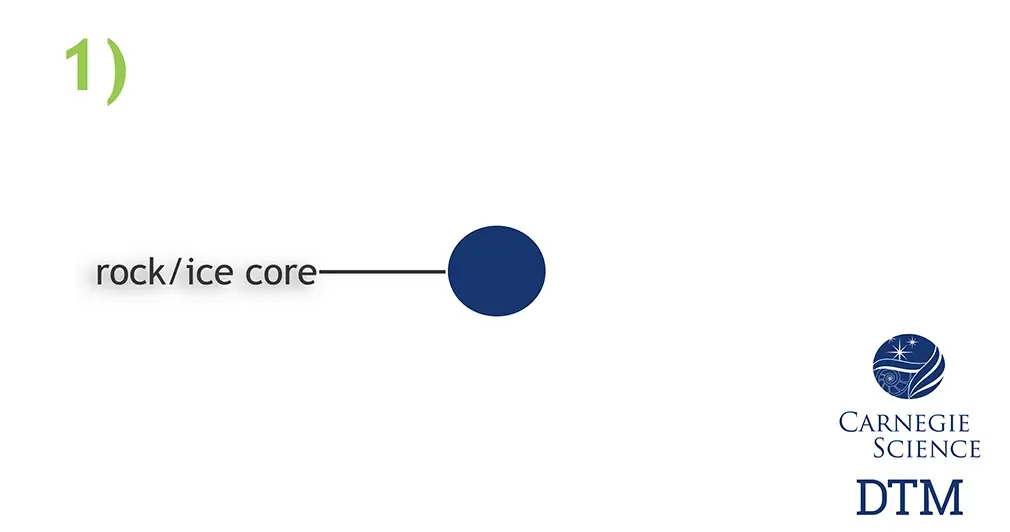
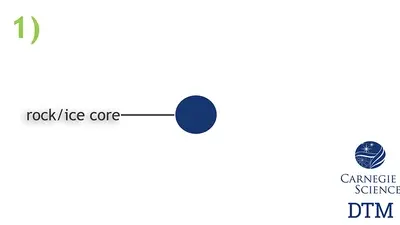
The formation of a gas giant
What might the findings in your paper tell us about our own Solar System?
If the model described in my paper turns out to be viable, it will help us understand how the gas giant planets of the Solar System formed, and how and when giant planets form elsewhere. It may also help us to understand planet formation more generally, since we now have a fairly good estimate of what fraction of stars host giant planets to compare with models. Ultimately, this might help us understand why many other planetary systems seem different than our own (with planets intermediate in size between Earth and Uranus orbiting close to their star), and how often Earth-like planets may form.
Why do scientists build models to try to understand how our Solar System formed?
Working out how planets form is very challenging. The Sun's planets formed very long ago, and much of the evidence for how this happened has been lost. Other planetary systems are forming today, but they are very far away, and it's hard to see them in detail. Models can help fill in some of these gaps by using what we know about physical and chemical processes and seeing what sequence of events can lead to the kind of planets that we see.
A lot of people are interested in where we come from, how life arose, the places where life can exist, and where else we could find it. One step towards answering these big questions is to understand how planets form and acquire their characteristics. We live on a planet, and as far as we can tell, planets are good places to look for life elsewhere. But some planets are more hospitable than others in terms of features such as their climate, atmosphere, or the presence of oceans. Knowing how planets form can help us understand why they are so varied and what makes them habitable.
How reliable are these models?
Planet formation turns out to be a very complicated process, and unfortunately there are still some fundamental issues that we don't understand. For example, lab experiments show how tiny dust grains can stick together to form objects the size of a pebble. But how these pebbles clump together to form much larger bodies, like the size of an asteroid, is still being studied. It also appears that the orbits of young planets can change dramatically in some cases, but we still don't know how often or how much the orbits change. Given these uncertainties, we should be cautious of drawing too many conclusions from models for planet formation.
Why is modeling the existence and characteristics of gas giants challenging?
There are several challenges, but probably the biggest one is modeling how a solid planet grows large enough to capture gas. We think that planets first form as solid objects composed of solid materials such as rock, metal, and ice. If a planet becomes large enough, it can capture an atmosphere from the surrounding cloud of gas in which the planets are forming. For planets the size of Mars or Earth, this atmosphere is relatively thin and represents a small fraction of the planet's total mass. Larger planets can gain thicker atmospheres, and above a certain mass, a planet will pull in more and more gas until something shuts off the supply. Examples of these are gas giant planets like Jupiter and Saturn. However, clouds of gas surrounding young stars don't last long—typically only a few million years. The problem is to form a solid planet that is large enough to pull in lots of gas while the gas is still available. This means the solid planet that is the seed for a gas giant has to grow very fast. To date, models have had a hard time explaining this very rapid growth.
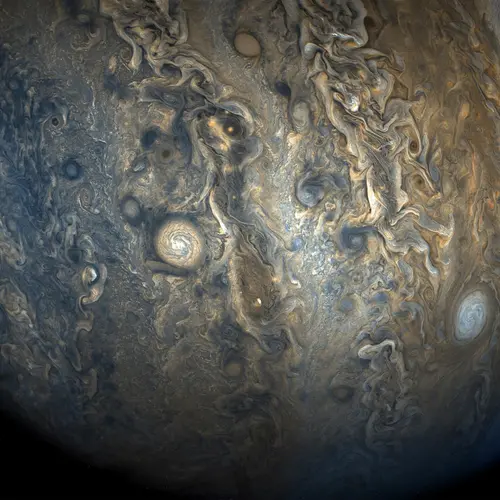
This image captured by the Juno spacecraft on October 24, 2017 shows stormy weather on Jupiter's southern hemisphere.
Credit: NASA/JPL-Caltech/SwRI/MSSS
What challenges does the core accretion model face in trying to explain gas giant creation?
The main challenge is that a solid planet has to grow substantially larger than Earth in order to pull in large amounts of gas and become a gas giant planet. The formation of gas giants has to take place within the lifetime of the gaseous protoplanetary disk surrounding a young star in which the planet is forming. Astronomers have surveyed nearby young stars to see which ones have these clouds of gas, and the answer is that only stars younger than a few million years have them. Older stars have lost their protoplanetary disks. So, solid planets have to grow large—and rapidly—if they are to become gas giants. In the Solar System at least, the giant planets orbit quite far from the sun. Planetary growth ought to have been slow here because the orbital speeds are slow, and planetary building blocks would have been far apart, so collisions leading to growth would have been relatively rare.
How do the size, makeup, and characteristics of the material in the protoplanetary disk affect formation of the solid core and gaseous components of a baby gas giant?
Traditionally researchers have assumed that the main building blocks of planets were asteroid-sized objects called planetesimals. How these objects formed is still uncertain, but they almost certainly existed. The Solar System contains a huge number of asteroids and comets. These are very diverse objects that formed early in the history of the Solar System, and we can think of these as leftover planetesimals that didn't get swept up by any of the planets. Unfortunately, computer simulations show that building planets from planetesimals is a slow process, and it is hard to explain how the core accretion scenario can work in this case.
Recently, researchers have found that planets would have grown faster if the main building blocks were pebble-sized objects. While a star's gaseous protoplanetary disk was still present, any pebble that passed close to a planet would have been slowed by drag as it moved through the gas. In many cases, this would have allowed the planet's gravity to capture the pebble and grow. If lots of pebbles were present, solid planets would have grown rapidly, allowing gas giant planets to form. Interestingly, recent observations of young stars suggest they typically have huge numbers of pebble-sized particles in orbit around them, which supports the idea that pebbles were important in planet formation.
What might the findings in your paper mean for future research and for DTM's Astronomy and Astrophysics?
This work should help refine the core accretion model for giant planet formation and improve our understanding of the role that pebbles play in planetary growth. If pebbles are important, then researchers will have to pay more attention to the nature and composition of planetary atmospheres as planets are growing.
In addition, having a better idea of how individual planets grow will make it easier to study the formation of entire planetary systems. A good deal of research (including my own) is now focused on modelling the possible diversity of planetary systems and comparing these models with the thousands of extrasolar planetary systems that we now know about.