A little over 50 years ago, the theory of plate tectonics emerged to provide a unifying theory for the dynamic behavior of the solid Earth as expressed by earthquakes, volcanoes, mountain building, and continental drift. Peter van Keken, a geophysicist at Carnegie's Department of Terrestrial Magnetism, studies the processes driving plate tectonics. Where does the energy driving these processes come from, and what are the consequences for both the immediate structure of the Earth and its long-term evolution?
On Thursday, March 22, 2018, van Keken will discuss these questions in the first installment of Carnegie's Spring 2018 Neighborhood Lecture Series. In preparation for his lecture, van Keken sat down for an interview touching on his research on plate tectonics and his work prior to joining DTM in January 2016.
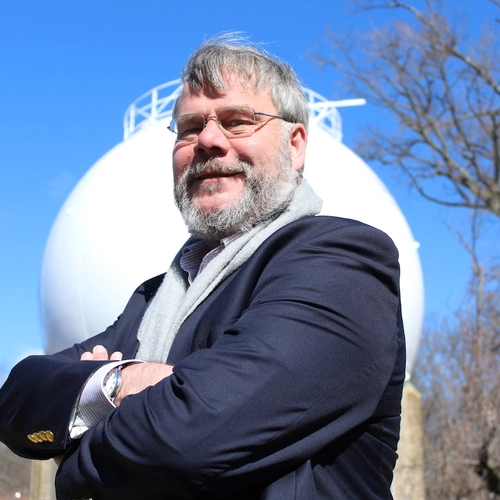
Peter E. van Keken joined Carnegie's Department of Terrestrial Magnetism as Staff Scientist in 2016 after 21 years as Professor of Geophysics at the University of Michigan. Photo by Roberto Molar Candanosa, DTM.
DTM: What is plate tectonics?
PvK: It's a 50-year-old theory that dramatically changed the way we look at the dynamics of the Earth. It was actually in a meeting in Washington, D.C., in 1967 where abstracts were submitted that all demonstrated, in independent observations, data that confirmed what we now think of plate tectonics: the Earth's surface is not static but is moving horizontally very slowly. One can think of the Earth as being divided into more than ten big plates that move independently of one another. These are places where you get earthquakes and volcanoes because of this interaction in locations such as around the Pacific "Ring of Fire". In some places, plates crash into each other. In other places, they move away from each other or slide along each other.
We think that our planet is the only one among the major planets with plate tectonics. We know that Mars and Venus do not have plate tectonics, or that at least they lack signs of plate tectonics such as mid-ocean ridges and trenches. In general, the fact that we have a liquid ocean on Earth is attributed to this uniqueness that as water is going down the Earth, it is actually helping lubricate the motion of the plates.
It is possible that some of the icy satellites of Jupiter and Saturn exhibit some form of plate tectonics. This is possible particularly for Europa, which looks like it has some form of little ice shelves that crash into each other and break apart similar to what we have on Earth's oceans where ice floats and icebergs are moving with respect to each other. Venus and Mars are very dry.
What has changed since the discovery of plate tectonics?
We know a lot better how plate tectonics operates on Earth. We understand how it shapes the Earth's surface a lot better. We understand how plate tectonics explain very large numbers of geological phenomena that, in the past, we explained by ad hoc processes that didn't necessarily make sense. Now we understand why geological phenomena like mountain formation and volcanoes form at the edges of subduction zones. That's significant progress that has been made by geophysical analysis, including seismology and geodetics. Just by using GPS instruments, we can actually see the plates move. We don't have to infer it from long-term observations. The reason we could not see it before is that the motion was very slow: a few centimeters per year. That's about how fast your fingernails grow. So, next time you clip your fingernails think about plate tectonics!
What is the ultimate goal of your research?
A real ambitious goal would be to fully understand the dynamics that drive plate tectonics. We see plates going down and we understand their current and past movements quite well. We just do not know fundamentally why it works.
It is much easier to explain the type of tectonics we see on Venus and Mars. For example, Venus doesn't really look like it has continents. Its surface is mostly basaltic crust, which is what we have on Earth under oceans. We don't know why the Earth has figured out how to break these plates and allow them to slide by each other. We think that by bringing water in, weaker minerals are created to help the plates slide past each other. But that is largely unknown in large part because these processes occur at depths that are way beyond what we can drill to. Everything is based on indirect observations from geochemistry and geophysics, and from rocks that sometimes come back after having been subducted. There are rocks in the subduction zones that go down to a depth of 80-100 kilometers (50-62 miles), and then for some reason come back up. They provide us with direct information about what is happening at the top of those plates.
What kind of projects are you working on as a DTM scientist?
One of them is directly related to subduction zones. My contribution to all of these projects is not to do the actual physical or chemical observations. Instead, I make model predictions of how the Earth should behave and then I compare them to the observations. Most of the time I'm wrong in my predictions, but that informs me on how to improve my models. This is work that is in collaboration with seismologists like Geoff Abers at Cornell University, petrologists like Brad Hacker at the University of California, Santa Barbara, and computational scientists like our own Cian Wilson—in addition to a number of postdocs and graduate students. We try to make better models of what a subduction zone looks like compared to observations and make improvements. Unfortunately, in that process we have to make a number of ad hoc assumptions, including how exactly these plates go down.
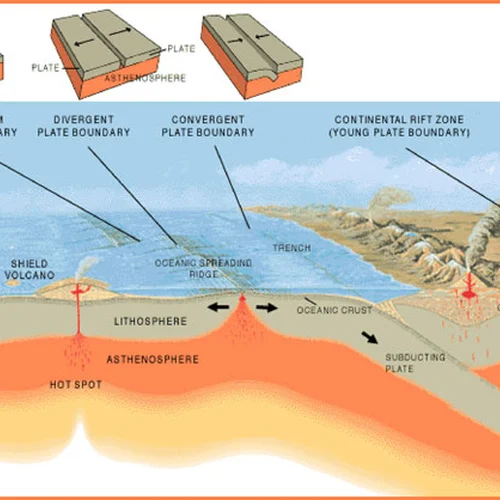
The three main types of plate boundaries. Image courtesy of the U.S. Geological Survey.
How do you come up with these assumptions?
We are helped by the fact that the Earth is governed by conservation of mass, conservation of energy, and conservation of momentum. We know how forces are balanced. We know that you can't create mass out of nothing and that you can't create energy out of nothing. That gives us the ability to describe the Earth in the form of differential equations that we can then solve using numerical methods.
What do you like about being a geophysicist?
The little successes we've had explaining things that were never before explained makes me excited. For example, one of the discoveries we made in 2002 was exciting. In order to make the type of magmas that come up in volcanoes, one does not require a large amount of friction, as had been assumed before. Frictional heating is attractive as a way to generate heat in models to explain phenomena (such as volcanism) that require heat. We all know we can rub our hands together to make them warmer, but it comes at a cost (of getting tired in the long run). It somewhat makes sense that frictional heating was invoked in the past. After all, a cold lithosphere is going down at the trench in a subduction zone, and very close to the trench are volcanoes. We were able to demonstrate that you can create those magmas with the characteristics that they have without any of the friction. It's just a natural consequence of the convection that occurs above a sinking slab along with the use of realistic deformation laws.
Another observation that amuses me about the Earth is that it can be represented as a solid and a fluid at the same time, based on what observations you use. On the one hand, the Earth is a solid, since seismic waves, including shear waves that only can be guided through solids, travel through it. It is therefore an elastic solid. But on long timescales, the Earth behaves as if it is a fluid; plate tectonics can be seen as the surface manifestation of sluggish convection in the Earth's mantle. With "long" here, I mean more than tens or thousands of years. One way to think about this conflicting behavior is to think of the Earth as an elastic solid in short timescales and a viscous medium in long timescales. The Earth is like silly putty. If you take silly putty out of its little egg, it's all oozed out. But you can take it and throw it against the wall, and it bounces all over the place. That's elastic. But then you let it sit, and then it oozes out. That's viscous. If you take the ball and stretch it out, it moves plastically. But if you snap it really quickly, it breaks. That's the brittle behavior the Earth has that causes earthquakes.
Are there things people tend to misunderstand about your research?
In more than two decades experience teaching undergraduates at the University of Michigan, I have found that many are enthusiastic about discovering where earthquakes and volcanoes come from. A typical question is whether we can predict earthquakes. The answer is pretty much no, or at least not yet. Can we predict volcanic eruptions? Yes, for the most part. We are getting much better at that, but we are helped by the fact that volcanic eruptions are preceded by significant precursors.
What was teaching at the University of Michigan like?
I particularly enjoyed teaching the introduction to geology course. Many students take it to satisfy their science curriculum requirements. But I was able to get a lot of students into geology, enough so that they became majors in the department. That made me very happy. One year I had 50 percent of the students become geology majors. There was a significant growth in the number of undergraduate majors we had, and I was happy to contribute to that.
My class was a normal lecture class. But in this course and others, I tried to make sure we had very engaging teachers that could tell the story in an engaging way. Teaching is all about communication. I have an interest in educating people, and you can do that by making your lecture more engaging and talking to the students more often. I often got to know the names and some of the backgrounds of many of the students.
What is the most challenging aspect of your particular niche of research?
The financial needs I have are for researcher's salaries and computational resources. I'm more concerned about finding the talent to do this kind of work. What I do requires an understanding of physics, chemistry, mathematics, numerical methods (to solve the mathematics), and the ability to apply it. I feel very fortunate that I can work here at DTM with very talented postdocs and staff. The postdoctoral fellow program we have here is fantastic.
The challenges are to understand how we can cast the planet dynamics into the differential equations that we need to solve and to understand how the "silly putty behavior" (short timescales for elastic motion and brittle motion and long timescales for viscous motion). How can we approach that interface between the two? That's eventually what will lead us to discovering why plate tectonics works.
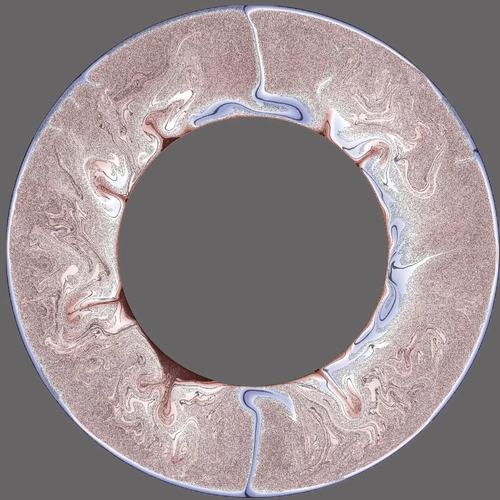
Mantle mixing calculations such as these allow for quantitative tests of scenarios that have been put forward to describe the chemical and thermal evolution of the Earth's mantle since its formation. Mantle convection in the Earth introduces heterogeneity through the subduction of basalt (black tracers) in subduction zones (blue regions). As this heterogeneity warms up it mixes back into the mantle but can also form dense piles near the core-mantle boundary (dark red regions). Inner circle is the core-mantle boundary; outer circle is the surface of the Earth. Image courtesy of Peter van Keken.
What are the toughest challenges of the scientific community?
I think the biggest challenges we have are to keep people healthier and safer, and to make sure we keep the planet habitable. Climate change certainly is a challenge. It will probably not affect me in my lifetime, but it certainly concerns me for many younger people, like those I was teaching at Michigan, and anyone's children and their children's children.
What's next for an established scientist like you?
I am ecstatic to be a part of the engaging and intellectual environment that Carnegie provides. To answer your question directly - this is it! There is absolutely no reason to go somewhere else. This place provides me with the colleagues and the resources that allow me to do my research.
Other than your research, what else gets you excited?
Languages! I'm just intrigued by language. As a kid, I was very good at languages, so now my hobby is to try to understand how languages work. I made a joke the other day here on campus that my goal in life is to be able to read Harry Potter (or more serious literature) in Japanese.
I speak English, Dutch, and French. I also speak adequate German. But Japanese is so incredibly hard. It's a language that's built on entirely different principles than English or Roman languages. Every time I learn something new, I realize how hard it must be for the Japanese to learn English.
What are you going to talk about during your Neighborhood Lecture?
It will be a combination of what you might see in an introduction to geology class, what you might read in a Scientific American article, and a combination of some actual scientific discoveries we've made. I'm going to talk about how plate tectonics was discovered, how we know about it, and how it affects us and the natural hazards we see on Earth.
---
Join DTM's Peter van Keken at 6:30 p.m. on Thursday, March 22, 2018 as he discusses how our thinking of plate tectonics has evolved, how natural hazards are connected to the slow convective motion of the Earth's interior, and how plate tectonics influences the long-term evolution of the Earth.
For event details and registration, visit: https://www.eventbrite.com/e/dr-peter-van-keken-presents-when-earth-attacks-causes-and-consequences-of-a-tectonic-planet-tickets-43401862138
—Roberto Molar Candanosa