EPL History
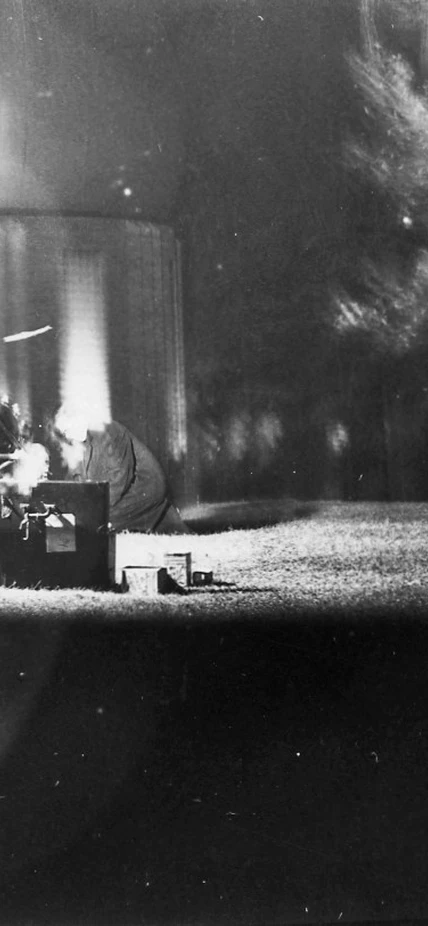
More than a century of science
The Earth & Planets Laboratory (EPL) traces its origins to the establishment of Carnegie’s Department of Terrestrial Magnetism (DTM) and Geophysical Laboratory (GL) in 1904 and 1905, respectively. DTM’s original mission was to investigate the magnetic and electrical fields of the Earth, while GL pioneered experimental studies of rock and mineral formation and the Earth’s interior.
In 2020, after three decades of sharing the Broad Branch Road campus and increasingly close collaboration, the two departments united to form the Earth & Planets Laboratory.
Over time, and through many historic moments in our country's history, the department’s research agendas evolved, contributing substantially to the rise of several new scientific disciplines as they grew, such as isotope geochronology, biogeochemistry, mineral physics, astrobiology, and the increasingly multidisciplinary study of exoplanets.
Throughout our history, both departments—and now EPL—subscribed to a multipronged and collaborative approach to science that encourages a mix of observational, experimental, theoretical, and computational research. Even as scientific goals change over time, it is this spirit that allows our researchers to freely tackle the most challenging questions about our planet and its place in the universe from all angles.
EPL History TimelineOur Founding Departments
By 1929, DTM researchers had collected volumes of data that were used to correct navigational charts and quantify the mysterious temporal variations in the geomagnetic field. The work was completed, and the department turned its attention to other questions.
During the 1930s and 40s, studies in physics dominated research at the department, which was a world-class center for nuclear physics. DTM investigators made fundamental discoveries about atomic forces. Throughout World War II, department scientists - like scientists all over the country - became involved in the war effort. One project, the largest after the Manhattan Project and the MIT Radiation Laboratory, was directed by Tuve. Under his guidance, researchers engineered an invention called the proximity fuze into mass production. The device was a radio-controlled sensor installed in the nose of an artillery shell. It detected a radio-reflecting target and triggered a detonation when the shell was in close proximity to its mark.
After the war, DTM physicists began some of the earliest work in biophysics using radioactive tracers. Later came ventures in seismology, astronomy, theoretical astrophysics, planetary formation and evolution, and radioisotope geochronology.
Science at the department has evolved to reflect the growing multidisciplinary nature of the Earth, planetary, and astronomical sciences. However, the historic goal—to understand the physical Earth and its place in the universe—remains a compelling beacon.
On December 2, 1901, Andrew Carnegie formally announced the future gift of ten million dollars for a scientific institution in Washington, DC.
On December 16, 1901, George F. Becker, the then director of the physical laboratory at the U. S. Geological Survey, submitted an outline for a geophysical laboratory entitled “Concerning the Geophysical Laboratory” to Charles D. Walcott, who was soon to be appointed Secretary of the Carnegie Institution's Board of Trustees. More detailed proposals were submitted the following year by the Advisory Committee on Geophysics for the Carnegie Institution of Washington and the "Committee of Eight." By the end of 1903, the Advisory Committee on Geophysics agreed on and published a set of specific plans for staff, building design, budget, and organization for the proposed laboratory. With almost every detail arranged and accounted for, the Trustees officially approved and established the Geophysical Laboratory of the Carnegie Institution of Washington on December 12, 1905.
Programs in volcanology, seismology, high-pressure research, and experimental petrology followed in the 1910s and '20s, driven by great contributions by such renowned Laboratory staff members as N. L. Bowen, H. S. Washington, and G. W. Morey. Crystal structure determinations using x-ray diffraction were initiated in 1919 under R. W. G. Wyckoff. Upon Philip Abelson's arrival as director in 1953, the Geophysical Laboratory broadened its investigations to include biogeochemistry. In the 1970s and '80s, a flourishing mineral physics program was begun. In the 1990s, its program expanded to areas of materials physics and microbiology, with a focus on extreme environments.
From 1906 to 1907, architects, designers, and construction workers labored tirelessly until the laboratory was finished at the Upton Street campus in northwest Washington, DC, in June of 1907. The total cost exceeded $300,000. Under the watchful eyes of Director Arthur L. Day, the Geophysical Laboratory flourished and rapidly established a reputation for excellence in the physical-chemical studies of rocks and minerals. This tradition of excellence has proved a trend over the last hundred years.
In 1990, the Geophysical Laboratory co-located with Carnegie's Department of Terrestrial Magnetism on its current site on Broad Branch Road in Northwest Washington, D.C. The move proved advantageous, facilitating cooperation between the Department of Terrestrial Magnetism and Geophysical Laboratory scientists for experimental and theoretical purposes.
In 2020, the Geophysical Laboratory merged with the Department of Terrestrial Magnetism to form the Earth and Planets Laboratory to further cement this cooperation and collaboration.
Our History by Category
In the early 20th Century, researchers from the Department of Terrestrial Magnetism (DTM) fanned out across the globe to conduct the first World Magnetic Survey. Involving more than 200 overland expeditions and ten ocean cruises between 1905 and 1944, the Survey aimed to answer fundamental questions about the origin of Earth’s magnetism and the electrical fields of Earth and its atmosphere. Valuable oceanographic data were collected, as well. In 1925, the first direct measurement was made of the height of the ionosphere. In the 1930s, DTM began coordinating cosmic-ray research worldwide.
Concurrent with DTM’s observational work, scientists at Geophysical Lab (GL) began applying physics and chemistry to understanding the origin of Earth’s building blocks in the laboratory, systematically investigating the structure and behavior of minerals at high pressure and temperature. Samples from active volcanoes, hydrothermal regions, and even the ocean floor were collected and analyzed to corroborate experimental results. Such studies necessitated the development of specialized instrumentation in-house – a tradition that continues at EPL today.
During World War II, efforts shifted to national defense projects, notably the development of the proximity fuse under Merle Tuve’s leadership. The fuze was one of the world’s first smart weapons and contributed significantly to the Allied victory. With the return of peace, new avenues of research were opened at both departments in the 1940s and ‘50s. Artificial earthquakes were created with explosives, and the resulting seismic waves were used to reveal crustal structure. Mass spectrometry was employed to determine the ages of minerals through the decay of radioactive isotopes. Cooperative research relationships were established across Latin America, beginning with Carnegie’s participation in the International Geophysical Year in 1957.
In the 1950s and ‘60s, under Philip Abelson’s leadership at GL, the gap between physical and life sciences began to be bridged through studies of organic processes in the Earth - biogeochemistry. By the 1970s and ‘80s, stable isotopes began to be applied to ecological and geological problems. The mechanism of subduction and the connection between crust and mantle were delineated. Mineral physics—a new field that links the observed chemical and physical properties of minerals to their geological behavior in the interior of the Earth and planets—began to take shape.
In the late 20th and now the 21st Century, access to high-performance computing brought previously unimaginable opportunities for exploring Earth’s interior through computational modeling. By integrating mechanics, seismology, geochemistry, and mineral physics, scientists in EPL’s computational geodynamics group seek to answer fundamental questions about the causes of plate tectonics and the evolution of Earth’s core and magnetic field.
Advanced computing and data science approaches have also opened a new frontier of data-driven discovery in mineralogy at EPL. “Mineral evolution” was introduced in 2008 as a way of explaining the diversity and distribution of minerals on the Earth and planets as a consequence of physical, chemical, and biological processes through deep time.
In 1911, Geophysical Lab (GL) scientists journeyed to Hawaii to collect lava samples and gases from Kilauea. It marked the start of more than a hundred years of EPL investigation into volcanoes, earthquakes, and the dynamic Earth. Early Carnegie grants supported pioneering volcanologist Frank Alvord Perret and enabled the publication of his milestone monographs on Vesuvius, Mt. Pelee, and Montserrat. In the 1920s, the first scientific drilling was conducted at Yellowstone National Park to explore the “plumbing” of its famous geysers and hot springs. As chair of Carnegie’s Advisory Committee on Seismology in the 1920s and ‘30s, GL’s founder Arthur L. Day played a pivotal role in catalyzing and organizing earthquake research in California.
The development of the broadband seismometer and borehole strainmeter at the Department of Terrestrial Magnetism (DTM) in the 1960s and ‘70s invigorated earthquake seismology and shed light on slow or “silent” earthquakes. In the 1980s, large arrays of portable seismometers began to be deployed around the globe, and cooperative networks organized to support such arrays. The most ambitious of these, the Kaapvaal Craton Project (1996-2002), brought together scientists from DTM, GL, and other American and South African institutions in a massive, integrated, geophysical and geochemical investigation of the ancient lithosphere of southern Africa.
In the 2010s, volcanoes once again became the focus of intense EPL geophysical study. Using new technologies such as quick-deploy seismometers and portable gravimeters, EPL scientists are now gaining new insights into patterns of volcanic eruption and the movement and evolution of magma in the crust.
The study of matter under extreme conditions has been one of EPL’s mainstays since its inception. Using furnaces and presses to mimic conditions found deep in the Earth, the first generation of Geophysical Lab (GL) scientists initiated systematic investigations of rock-forming minerals, applying them to understanding the formation and evolution of igneous rocks. Contributions by such renowned staff members as N. L. Bowen and G. W. Morey quickly established the Lab’s reputation as a global center for experimental petrology and was solidified by the landmark publication of The Evolution of the Igneous Rocks in 1928.
Throughout the 20th Century, new technologies such as diamond-anvil cells in the 1960s enabled ever-deeper regions of our planet to be probed in the laboratory. In 1975, a pressure of 1 million atmospheres – equivalent to that found in the lower mantle – was achieved by Peter Bell and H. K. Mao. A decade later, the 2-megabar barrier (corresponding to outer core depths) was broken. In the 1990s, collaborative agreements brought beamlines at national accelerator laboratories into the arsenal of instrumentation available to GL scientists. While originally focused on Earth materials, high-pressure investigations at EPL in the 21st Century are also being applied to synthesizing and characterizing many kinds of advanced technological and energy-related materials.
Understanding the properties of matter at an atomic scale has been another long-standing goal of research at Carnegie. As early as 1919, X-ray diffraction was first applied to crystal structure determination at GL. In the 1920s and ‘30s, using electrostatic generators to accelerate particles, Department of Terrestrial Magnetism (DTM) scientists began probing the structure of the atom. The most powerful of these, a 3-million-volt Van de Graaff accelerator that still towers over the EPL campus, was used in 1939 for one of the first demonstrations of uranium fission in the United States.
Since the 1990s, high-speed computers have been applied to theoretical materials research. First-principles calculations are used to predict many material properties under extreme conditions, complementing and verifying experimental results.
The serendipitous discovery of radio emissions from Jupiter by Department of Terrestrial Magnetism (DTM) astronomers in 1955 and the analysis of Apollo lunar samples at the Geophysical Lab (GL) in 1969 were early Carnegie forays into planetary science.
Still, Solar System research at Carnegie began in earnest in the 1970s, with DTM’s George Wetherill’s introduction of computer simulations to model how planetary systems form. By the 1980s, this work was joined by observational studies of star and galaxy formation (as well as the recognition, by Vera Rubin and colleagues, of the existence of dark matter in the universe). Laboratory analysis of the chemical composition of meteorites and other extraterrestrial material began in the 1990s. In the early 2000s, research expanded to asteroids, comets, moons, and the search for small bodies in the outer Solar System. In 2011, the MESSENGER mission, headed by DTM director Sean Solomon, became the first NASA spacecraft to reach Mercury in more than thirty years.
The turn of the 21st Century issued in a burgeoning era of exoplanet discovery and exploration at Carnegie, fueled by precision Doppler spectroscopy. Carnegie became a founding member of the NASA Astrobiology Institute in 1998 and joined the search for signatures of life on other planets and studied the emergence and evolution of life on our own.
Studies of exoplanet diversity at EPL reveal new insights into exoplanet formation, diversity, and habitability.
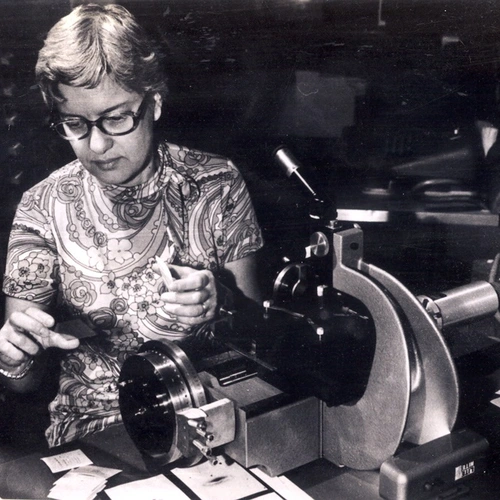
Discovering dark matter
After observing dozens of galaxies by the 1970s, Vera Rubin and her colleagues found that something other than the visible mass was responsible for the stars’ motions. Each spiral galaxy is embedded in a “halo” of dark matter—material that does not emit light and extends beyond the optical galaxy. They found it contains 5 to 10 times as much mass as the luminous galaxy.
As a result of Rubin’s groundbreaking work, it has become apparent that more than 90 percent of the universe is composed of invisible material—dark matter and dark energy.
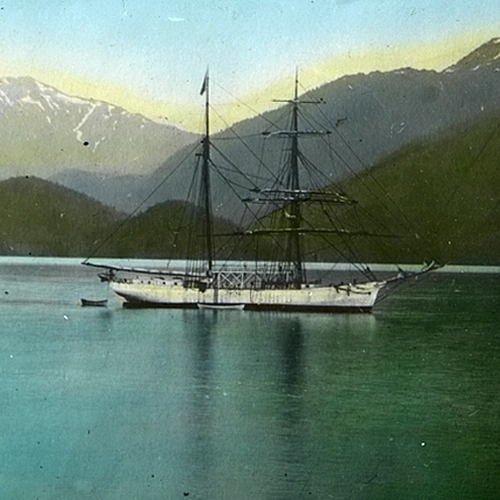
First world magnetic survey
At the turn of the 20th Century, scientists knew that the earth's Earth's magnetic field varied across the globe and over time, causing compasses to go awry and point as much as several degrees away from true north. Sailors found this inconvenient, but it was the scientific community that was truly perplexed.
The Department of Terrestrial Magnetism—one of the original departments that now make up the Earth & Planets Laboratory—was established by the Carnegie Institution of Washington in 1904 expressly to investigate the magnetic field of the earth and its variation, both over space and over time. Two vessels were employed in this investigation, first the Galilee, then the custom-built non-magnetic Carnegie.
Learn moreHistoric Publications
Explore turn-of-the century science through the historic Department of Terrestrial Magnetism (DTM) monographs in geomagnetism, atmospheric electricity, and more.
Four volumes of the Annual Report of the Director, Geophysical Laboratory were published from 1988 through 1991.
Highlights from our collection of 5,000 images of instruments and apparatus used in early 20th Century geophysics, atomic physics, and astronomy.
Discovery publications from EPL scientists.
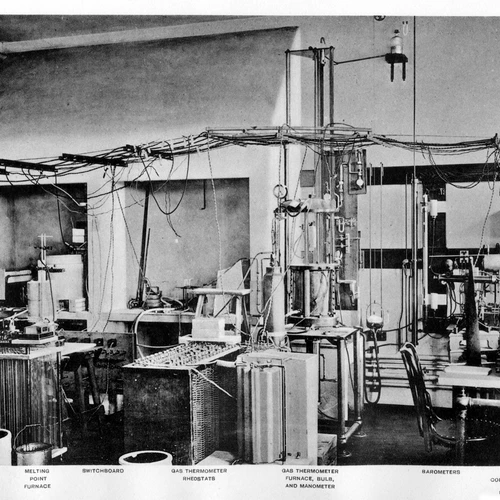
Establishing experimental petrology
Experimental petrology owes much to the foundation of the Geophysical Laboratory in 1905.
The Laboratory was established at a time when top igneous petrologists wanted to gain “exact science” prestige for their field—like chemistry or physics. However, while a respected area of geoscience research today, experimental petrology was largely ignored and unappreciated until the 1940s.

The proximity fuze and WWII
During World War II, our efforts shifted to national defense projects, notably the development of the proximity fuze under Merle A. Tuve’s leadership.
The proximity fuze revolutionized warfare for the United States during World War II. The fuze used Doppler radar to allow an artillery shell to detonate when it was near its intended target. This allowed for more precise targeting and optimal effectiveness. Until the development of the proximity fuze, a detonation relied on physical contact, a timer, or an altimeter.
Learn more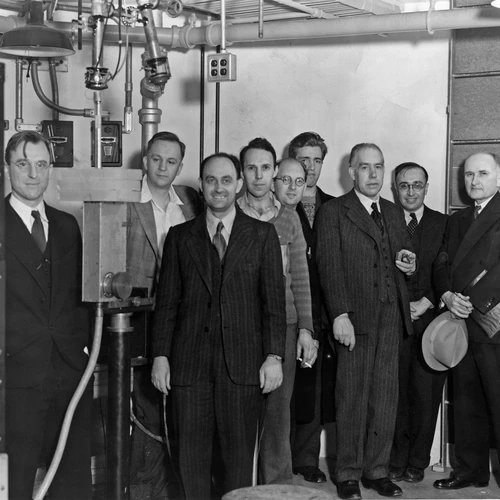
Smashing the atom
On January 28, 1939, our campus became one of the first places in the United States to demonstrate uranium fission. Only two days earlier, news of fission became public knowledge at the Fifth Washington Conference on Theoretical Physics, sponsored jointly by the Carnegie Institution and George Washington University. The experiment demonstrated it as a nuclear as well as a radio-chemical phenomenon. As it happens, Niels Bohr, Enrico Fermi, and other conference attendees were present to witness this historic event.
(Left to right: Robert Meyer, Merle Tuve, Enrico Fermi, Richard Roberts, Leon Rosenfeld, Erik Bohr, Gregory Breit, and John Fleming.)
Historic Memoirs
1865 - 1932 | Louis Bauer was the first director of the Carnegie Institution’s Department of Terrestrial Magnetism, from 1904-1929.
1877—1956 | John Fleming was director of DTM during World War II until 1946. He was in charge of all work under war contracts of the Institution concerned with ordnance devices, including proximity fuses. He devoted his life to the study of terrestrial magnetism and atmospheric electricity.
1901-1982 | Director of DTM from 1946–66. Developed the proximity fuse. Conducted first use of pulsed radio waves in the measurement of layers in the ionosphere
1925 - 2006 | George Wetherill was a physicist and geologist who served as the Director of DTM from 1975-1991. He originated the concept of the Concordia Diagram for the uranium-lead isotopic system.
1869–1960 | Arthur Day’s became GL's first director in 1907. His research spanned the fields of physics, physical chemistry, seismology, volcanology, and glass and ceramic research.
1887—1969 | Leason Adams was a physical chemist who became the director of the Geophysical Lab in 1936. He led the department through WWII.
1913 - 2004 | Philip Abelson joined DTM in 1939, became the director of GL in 1953, and then the President of Carnegie in 1971.
1921–2003 | Hatten Yoder began his 55-year association at GL in 1948 as an experimental petrologist. He was appointed director in 1971.