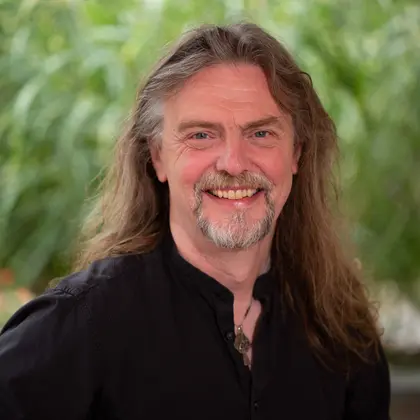
Q: You are part of the science team for the Mars Perseverance Rover, which recently announced the discovery of a very interesting rock on our neighboring planet. Could you tell us a little bit about what the rover found and why it has scientists’ attention?
Andrew Steele: Perseverance has been roving around inside Mars’ Jezero crater for a while and now it’s moved up to the rim. And as it was wandering around, it found a rock with a very interesting texture. And when the rover analyzed this rock, it started to pick up a lot of interesting sulfur minerals. And this grabbed our attention for several reasons. One, on Earth, living organisms reduce sulfur into sulfides like those seen on the Martian rock in order to generate energy. So, that’s very enticing to astrobiologists like me. Also, the calcium sulfate that we see on this rock is usually water deposited. So, it’s showing us that water was involved in the chemistry that was occurring on this rock, and we know water is crucial for our understanding of planetary habitability. Lastly, we picked up signs of organic carbon, which is also considered essential for life. What this tells us is that we have a pool of organic carbon in a mineralogical context that is extremely interesting. All of these signs have been seen in Martian meteorites associated with non-life processes, but on Earth they are known to be associated with life-derived processes as well.
Q: You’ve been studying organic materials in meteorites that originated on Mars for a long time. How does what the rover has found on this rock relate to your own work?
Andrew Steele: I have been looking at this phenomenon for 20 years in Martian meteorites—rocks that were ejected from the Red Planet and traveled all the way to us here on Earth. In my lab, I have been using the same techniques as Perseverance. We've picked these same carbon signals up previously, in little, tiny little bits in a couple of samples. What does this organic carbon mean? Well, in the meteorites it shows that Mars could do organic chemistry that is not powered by living organisms and synthesizes organic compounds that could lead to life or, indeed, be a food source for any life that did exist. We know that non-life processes can make this carbon and, in fact, the sulfates and the sulfides that we found, too.
Q: So, as an astrobiologist, why does finding potential evidence of non-life chemistry on Mars excite you?
Andrew Steele: We are very interested in understanding the geologic conditions and mineralogical interactions that could have transitioned between non-life chemistry and biochemistry on Earth. But the record of how life started on Earth is almost completely erased by the proliferation of life and the geologic process that recycle planetary material here. And what Mars gives us is a potential second insight into the processes that could form life in a much more pristine context. So, what we have in this rock is a juxtaposition of habitability—with the evidence of water—and, with the sulfur minerals—material that aligns with complex organic material that we believe was synthesized on ancient Earth.

Q: So, what does this mean regarding the search for evidence of life on other worlds?
Andrew Steele: When it comes to these sorts of investigations, I keep a null hypothesis in mind in which I always assume there is no life and I try to disprove that hypothesis based on the evidence of chemistry that we can see in a sample. When you’re looking for evidence of life, you want to see something that stands out and is different from an abiotic background chemistry that occurred in an environment.
Think of it this way, life doesn’t necessarily use all the material available to it. For example, there are lots of different amino acids found in a famous meteorite called the Murchison Meteorite, which fell in Australia in the 1970s. But we know that life chooses a subset of those building block materials—20 essential amino acids—and concentrates them. So, to look for evidence of life, you need to look for a concentration above the abiotic background and try to determine what it is that’s causing that concentration to occur. For example, instead of A,C,T,G a putative Martian organism may use L,M,N,O,P—a different sequence of letters but not the whole alphabet. This means that understanding the abiotic chemistry that historically happened on Mars will help us to establish that background and look for something really exciting. And rocks like this one, as well as the meteorites I’ve studied, can help us accomplish that.
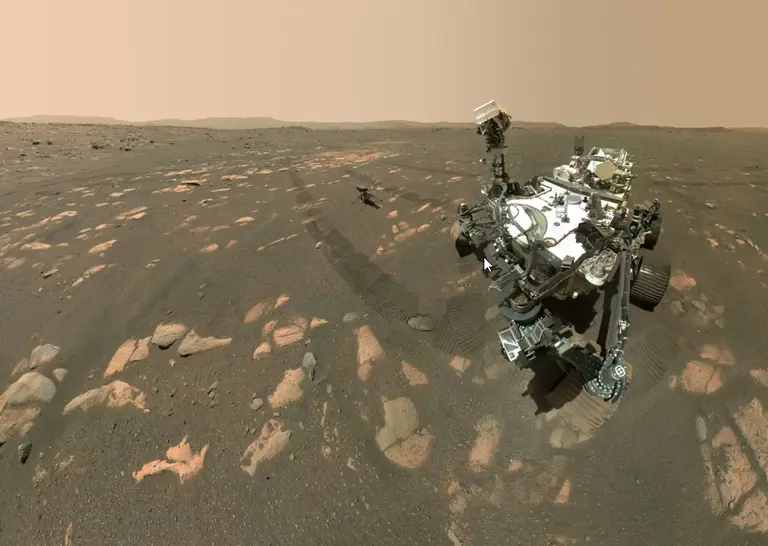
Q: What’s next in terms of studying this exciting rock find?
Andrew Steele: Rovers are brilliant. They're amazing stuff. They're some of the most complex spacecraft we've ever flown with the most incredible capabilities. But there are limits in the kinds of instruments that can be flown to another planet. This is why sample return is crucial for the next big breakthroughs in this field. Perseverance has set aside this rock for a future sample return mission, which would enable us to analyze it in much greater detail here on Earth.
Studying samples in Earth labs, we would be able to do so much more in order to understand and differentiate organic carbon that could have formed life and didn’t, from organic carbon that represents a remnant of life. This is an incredibly important distinction, and we need sample return in order to answer it. The record of how life started on Earth has been erased by our active planet. If these samples don’t contain the signatures of life but do have the signature of reactions that could lead to life, then that is really important as it would show us how life on Earth could have formed. So, these analyses don’t just answer the question “are we alone?” but also directly address the question “where did we come from?”
Q: How can sample return and the work that Perseverance is doing be applied to other planetary bodies?
Andrew Steele: Going to Mars takes about seven months, which is relatively easy compared to most other places where we would be interested in looking for evidence of life, or conditions that could indicate habitability. One of the things we will learn from these samples is how to tailor the next generation of instruments for missions that will go even further out into the Solar System, such as to Saturn’s moon Enceladus or Jupiter’s moon Europa. Mars sample return missions will enable us to know how to do life-detection properly and these lessons can be applied to exoplanet research as well.
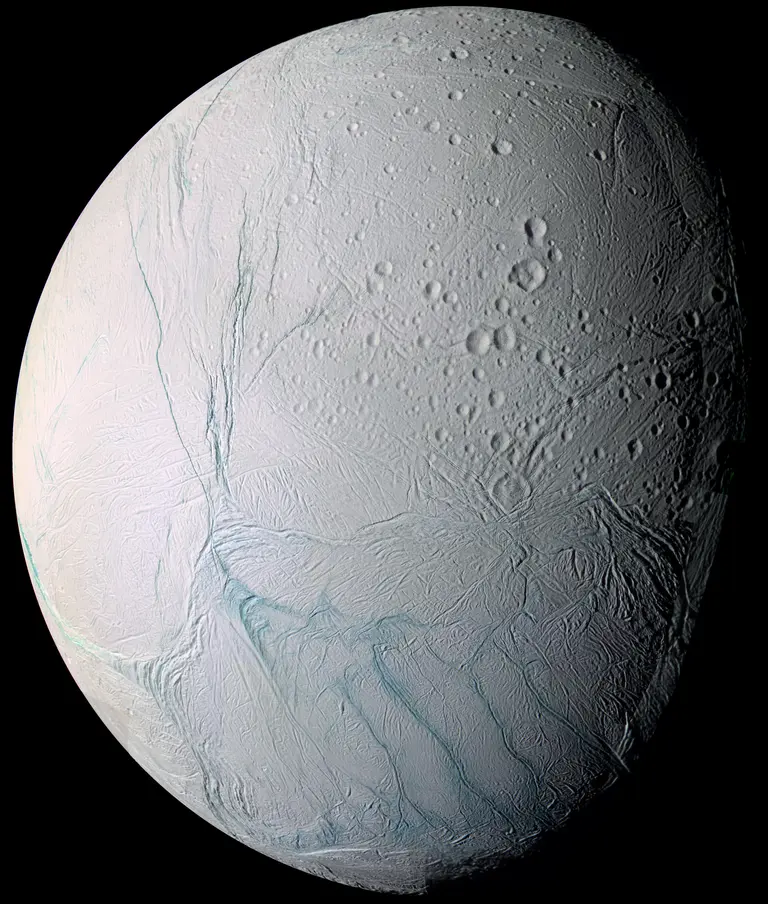