Baltimore, MD—Antibiotics can make easy work of infections. But how do they affect the complex ecosystems of friendly bacteria that make up our microbiome?
“When a doctor prescribes antibiotics, it sets up a multi-faceted experiment in your gastrointestinal system,” explains Carnegie’s Will Ludington “What can it teach us about the molecular principles of species interactions in nature?”
New work led by Ludington and Stanford University’s K.C. Huang set out to answer this challenging question and discovered a new form of antibiotic tolerance. Their findings, which have important health implications, are published by eLife.
This is one of several research fronts on which Ludington uses the fruit fly microbiome to understand interactions between species in a bacterial community. It poses an ideal environment for probing both natural bacterial populations and the human microbiome.
The human microbiome is an ecosystem of hundreds to thousands of microbial species living within our guts. It affects our health and even our longevity. But it’s difficult to elucidate the myriad ways that the different species that comprise our microbiome interact with and influence each other, even under normal conditions. Once antibiotics are introduced, little is understood about how these vital communities are impacted on a biochemical level.
This is why the fruit fly makes such an excellent model. Unlike the human microbiome, it consists of only a handful of bacterial species.
“We really wanted to understand how an antibiotic’s targeting of specific physiological processes impacts the metabolic interactions and sharing of resources that occurs between bacterial species within a community,” said lead author Andrés Aranda-Díaz of Stanford. “This is especially important because in nature bacteria live in diverse communities.”
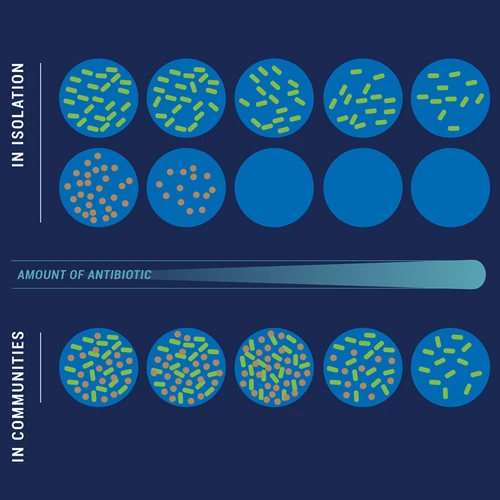
Image: The efficacy of antibiotics often differs between the lab and clinic. Interactions with other bacteria in nature can change the susceptibility of certain species to antibiotics. Here the orange bacteria can tolerate higher antibiotic concentration when grown together with the green bacterium (bottom) than when grown in isolation (top). Each blue circle represents a culture grown with a different antibiotic concentration. The antibiotic concentration increases from left to right. Image is courtesy of Navid Marvi and Andres Aranda-Diaz.
The simplicity of the fruit fly microbiome makes it the perfect vehicle for revealing how this multi-species biochemical interplay is altered by the introduction of antibiotics.
“We found that interactions between species in the gut microbiome ecosystem influence the effectiveness of antibiotics at killing off an individual species within this community, as well as the entire community’s metabolism,” said Huang.
The researchers demonstrated that when a type of bacterium from the fruit fly microbiome, called Lactobacillus—which are also found in yogurt—is grown together with a vinegar-producing fly bacterium called Acetobacter, it is less susceptible to death by antibiotics.
This is a newfound category of a phenomenon called antibiotic tolerance, meaning that cells die much more slowly when found together than they would on their own. Tolerance can be dangerous, because this delay increases the risk that full-on resistance to the antibiotic could evolve.
“Normally, tolerance occurs when a cell slows its metabolism in response to antibiotic exposure,” explained Ludington. “But in this case, the tolerance is actually associated with increased metabolism.”
It turns out that the Acetobacters consume the lactic acid that is excreted as a waste product by neighboring Lactobacillus, providing a fitness advantage to both species and triggering the tolerance the team discovered.
“We don’t know exactly how it happens yet, but we think the two bacterial species both ‘know’ when the other type of cell is there and respond appropriately,” said Benjamin Obadia of UC Berkeley. “These mechanisms are probably evolved from living together, and we wouldn’t have seen them if we studied the two species in isolation.”
The team’s work shows that the microbiome can be an important tool for understanding the relationships within communities of bacteria in the natural world on a biochemical level.
“It also illustrates that gut microbiome health should be considered whenever antibiotics are prescribed,” added Ludington.
Studying the principles governing species-species interactions are key to understanding so much about ecosystems large and small and the microbiome is a critical tool for exploring these questions.
Another recently published collaboration between Ludington, Huang, and a different Stanford researcher—biologist Lucy O’Brien—developed new technology to visualize the guts of living fruit flies. Called Bellymount, it allowed them to observe individual bacterial cells in the gut of a living fruit fly for the first time.
“By observing the microbiome in real time, we were able to measure its dynamics,” said Ludington of their paper, which appeared in PLOS Biology.
The research team found that specific regions of the gut have high microbiome stability and others have continuous turnover. This indicates that there are structures in the fruit fly gut that maintain colonization and opens the door to the possibility that fruit flies may have evolved these structures to keep their microbiomes.
“Now we have the power to actually eavesdrop on the ‘conversations’ occurring between microbiome bacteria, and the gut cells in their surrounding environment,” said Huang.