How Light Drives Photosynthesis—A New Model
Photosynthesis captures the energy in sunlight and traps it in chemical bonds. This process powers Earth’s biosphere and plays a critical role in how the planet operates. To achieve food and water security for a growing population, to transition to a carbon neutral energy system, and to forecast the dynamics of the carbon cycle and climate, scientists need to better quantify planetary-scale photosynthesis. To date, most approaches have relied on atmospheric carbon dioxide (CO2) concentration measurements. However, because CO2 is the product of respiration, the respiratory CO2 release obscures photosynthetic CO2 uptake.
To move forward, researchers need global measurements of a signal that is uniquely linked to photosynthetic activity and a model that explains the relationship between that signal and the photosynthetic process. Recently, satellite-based sensors obtained the first global measurements of a signal that is uniquely linked to photosynthetic activity: solar-induced chlorophyll fluorescence. Now, research associate Jennifer Johnson and senior staff scientist emeritus Joe Berry have developed a model that describes the quantitative relationship between chlorophyll fluorescence and photosynthesis.
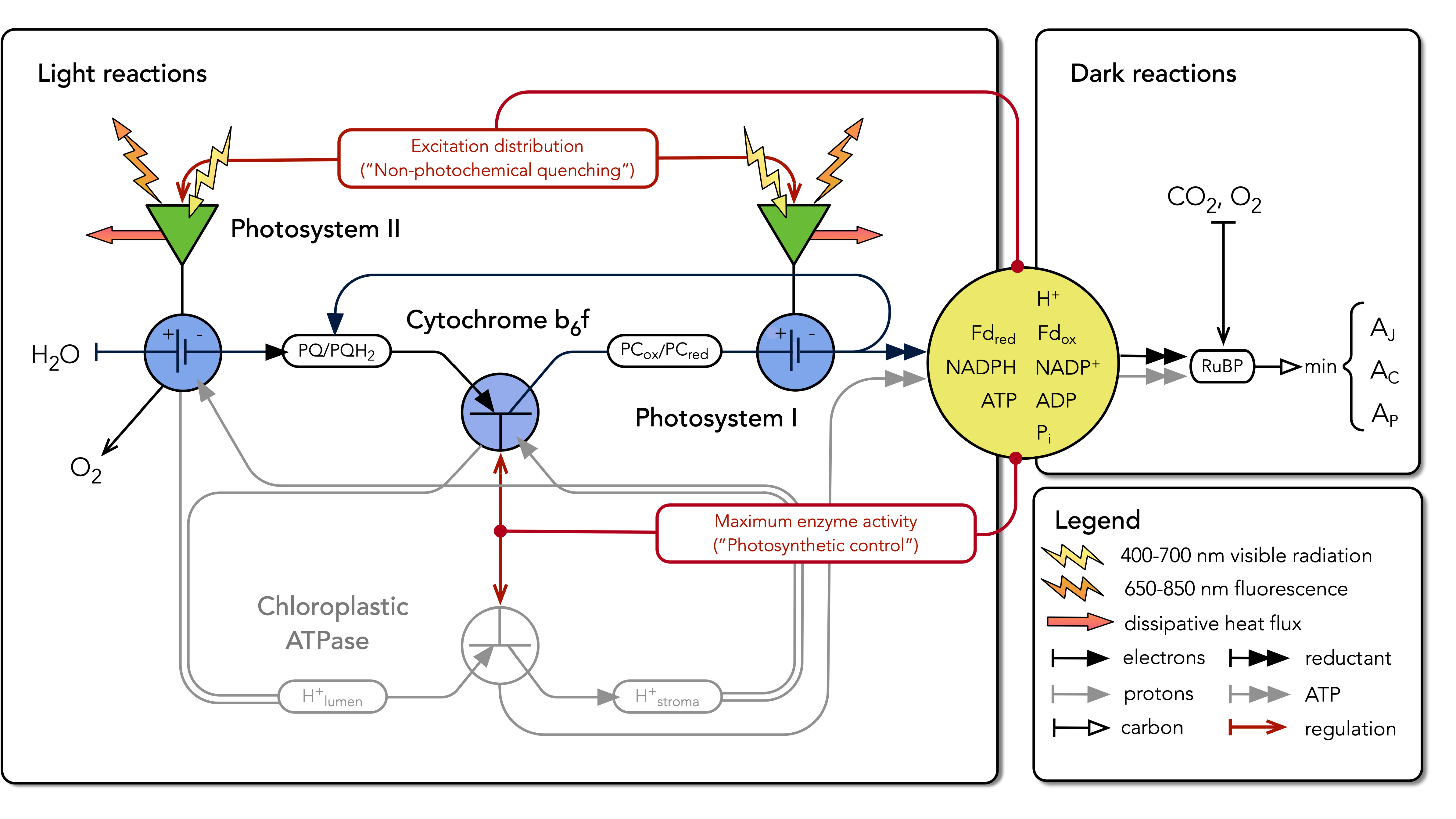
Jennifer Johnson’s new model treats the photosynthetic electron transport system as an electrical circuit.
The flow of electrons from water to reductant is viewed as a light-driven current. The speed of the current is primarily controlled by the availability of light relative to the activity of the enzyme called the cytochrome b6f complex (cyt b6f). When the availability of light is low, cyt b6f presents minimal resistance to electron flow, such that the speed of the current is proportional to the availability of light. When the availability of light is high enough that the speed of electron flow could exceed the system’s ability to use electrons, feedback from carbon metabolism increases the resistance presented by cyt b6f. This controls the flow of electrons so that they can be safely used. In this way, the regulation of cyt b6f both permits efficient photosynthesis and protects the plant from damage from too much light. By expressing these concepts quantitatively, the new model can simulate the carbon dioxide uptake and fluorescence emission associated with photosynthesis over the range of environmental conditions in nature.
In photosynthesis, the chlorophyll pigment absorbs light, and the photons are either trapped by photochemical reactions, dissipated as heat, or re-emitted as fluorescence. Then, the products of the photochemical reactions are used to fix CO2 into sugars. While much has been learned about the molecular details of each individual step, the overall coordination between the energy-producing and energy-consuming processes is not well understood. By exploring this relationship in laboratory experiments with single leaves, Johnson and Berry made a key discovery: the speed and efficiency of photosynthetic light capture are largely controlled by a single enzyme, the cytochrome b6f complex. The team translated this insight into a model that describes the overall photosynthetic process like an electrical circuit—and quantifies the relationship between fluorescence emission and CO2 uptake.
Traditionally, Earth system models have represented photosynthetic CO2 uptake, but not chlorophyll fluorescence. By updating their photosynthesis schemes to Johnson and Berry’s new equations, Earth system models can simulate both of these “fingerprints” of photosynthetic activity. Then, the models can use satellite-based measurements of chlorophyll fluorescence to quantify photosynthetic CO2 uptake in a way that is insensitive to respiratory CO2 release. Since photosynthesis is the single largest flux in the carbon cycle, improved quantification is expected to significantly improve understanding of carbon-climate feedbacks and predictions of future carbon cycle and climate dynamics.
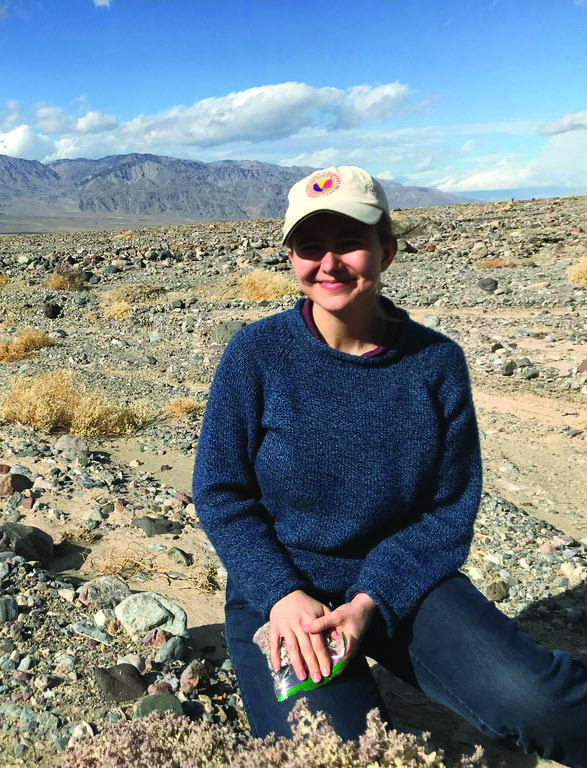
Jennifer Johnson is a research associate in Joe Berry’s Global Ecology lab. She investigates processes that control the exchange of energy, water, and carbon dioxide between the terrestrial biosphere and the atmosphere, using a combination of measurements and models. Image courtesy Carnegie Institution for Science